Introduction
Atherosclerosis is the main cause of cardiovascular diseases [1]. Although the exact etiology of atherosclerosis is unclear, it is generally recognized that it is associated with a variety of problems, including endothelial dysfunction, lipid buildup, and chronic inflammation [2, 3]. By generating endothelial cell (EC) dysfunction, recruiting monocyte-derived macrophages, and fostering chronic inflammation, low-density lipoprotein (LDL), particularly its oxidized form (ox-LDL), plays a critical role in the onset and progression of atherosclerosis [4]. The endothelium is the blood vessel’s innermost cell layer, and these cells form a particular barrier that separates blood from extravascular tissues. Recent research suggests that endothelial dysfunction in blood arteries plays a key role in the onset of atherosclerosis [5]. The fundamental processes, on the other hand, are little known.
Long non-coding RNAs (lncRNAs) are non-protein-coding RNAs that are longer than 200 nucleotides [6, 7]. LncRNAs have been confirmed to play a key role in an increasing number of diseases [8–10]. Although many lncRNAs have been found to be involved in atherosclerosis, compared with coding molecules, a relatively small number of genes has been studied. Atherosclerosis has recently been linked to the progression and prognosis of a handful of lncRNAs. These findings could shed light on the molecular mechanism underlying the disease [11]. The lncRNA GAS5 has been linked to an increased risk of atherosclerosis, and another study found that the lncRNA H19 affects the NF-κB and MAPK pathways, contributing to atherosclerosis [12, 13]. Additionally, prior research has shown that the lncRNA ENST00113 stimulates atherosclerosis cell growth and metastasis via the PI3K/Akt/mTOR pathway [14].
In human umbilical vein endothelial cells (HUVEC), hundreds of lncRNAs were found to be dysregulated in response to oxidized low-density lipoprotein loading in a recent study [4]. In our previous, we found that the lncRNA GAPLINC plays an important role in angiogenesis in HUVEC through targeting miR-211, and this lncRNA has been predicted to target another miR-183-5p [15]. More interestingly, the miR-183-5p has been shown to suppress apoptosis and promote proliferation in cancer cells by targeting programmed cell death 4 (PDCD4) molecules [16–18]. As a result, we hypothesized in this study that GAPLINC could be induced under high ox-LDL conditions and act as a decoy to sequester miR-183-5p and prevent it from binding to its target PDCD4, resulting in cell apoptosis.
Material and methods
Cell culture
Human aortic endothelial cells (HAECs) (Sciencell, America) were grown between passages 6 and 10; cells were cultured at 37°C with 5% CO2 in EGM-2 Bullet Kit media according to the manufacturer’s instructions (Lonza).
Qualitative PCR
Total RNAs were extracted using TRIzol Reagent. The Nono100 instrument was used to determine the RNA concentration and purity. Hiscript Reverse Transcriptase (VAZYME) and random hexamer primers were used to make the cDNA. The qPCR reaction was conducted using the SYBR Green reagent at 50°C for 2 min, 95°C for 10 min, followed by 40 cycles of 95°C for 30 s and 60°C for 30 s. The comparative Ct method was used to calculate the relative expression of each mRNA or miRNA. The GAPDH mRNA was used as an internal control. The experiments were carried out on using an ABI QuantStudio6 (Applied Biosystems).
Construction of adenovirus expression vectors
GAPLINC overexpression and silencing were accomplished with adenovirus vectors, as described in our prior study [15]. Briefly, the GAPLINC fragment was digested and ligated into pBF-adshuttle-GAPLINC overexpression vectors. The GAPLINC RNAi sequence (CCTGAAATAATGAACTCCT) was ligated into the pG1.1 plasmid. Both vectors were transfected into HEK293 cells to make the adenovirus vector.
Protein extraction and western blot analysis
Cells were washed twice with cold PBS buffer (0.01 mol/l, pH = 7.2) before being mixed for 30 min on ice with 400 ml of 1 mmol/l PMSF lysis buffer. Centrifugation at 3800 g for 5 min yielded the proteins. The BCA method was used to determine the protein concentration. SDS PAGE was used to separate protein samples, which were then transferred to a PVDF membrane. GAPDH (1 : 1000, Goodhere), Bcl2 (1 : 1000, Proteintech), and PDCD4 (1 : 2000, Boster) primary antibodies were incubated with the membrane overnight. The membranes were then incubated for 2 h at room temperature with secondary antibodies (1 : 5000). Finally, BandScan was used to analyze the data.
Cell counting kit (CCK)-8 assay
The manufacturer’s instructions were followed when using CCK-8. HAECs were inoculated into multi-well plates (5 × 103 cells/well). Briefly, CCK-8 solution was mixed with serum-free medium, and 10 µl of the mixture was added to each well. Cells were then incubated for 4 h at 37°C with 5% CO2, after which the optical density at 450 nm was measured.
Apoptosis and cell-cycle assays
FITC Annexin V Apoptosis Detection Kit I (BD Biosciences) was used to double-stain cells for the apoptosis assay, as per the manufacturer’s protocol. Cells were fixed in 70% ethanol, washed in PBS, and treated with RNase A (100 µg/µl) and IP for cell-cycle analysis. A flow cytometer (BD Biosciences) was used to assess stained cells immediately. All assays were performed in triplicate.
Dual-luciferase reporter assay
Firstly, GAPLINC wild-type (WT)/mutant (MUT) and PDCD4 3′untranslated region (UTR) WT/MUT were constructed. Then, using miR-183-3p mimics or NC mimics, they were co-transfected into HEK293T cells. A dual-luciferase reporter assay system (Donghuan Biotech) was used to determine luciferase activity 48 h after transfection.
TUNEL assay
According to the manufacturer’s instructions, TUNEL staining was used to assess cell apoptosis. Briefly, cells were fixed with 4% formaldehyde and then stained with a TUNEL kit. Fluorescence microscopy was used to count TUNEL-positive cells.
Results
GAPLINC level was up-regulated after ox-LDL treatment
Previous studies have shown that a higher level of ox-LDL can inhibit proliferation and induce cell death in endothelial cells [19, 20]. Therefore, we first treated HAECs with different concentrations of the ox-LDL. The results indicated that the ox-LDL significantly inhibited cell proliferation (Figure 1 A) and led to cell apoptosis, which is consistent with previous studies (Figure 1 C). We next treated HAECs with a high concentration of ox-LDL for 24 h and 48 h, respectively. Our results demonstrated that the longer time of treatment significantly inhibited cell proliferation (Figure 1 B) and induced cell apoptosis (Figure 1 D). We found that ox-LDL treatment inhibited cell proliferation and induced cell apoptosis in a time- and dose-dependent manner. Therefore, we next tested the expression changes of lncRNA, GAPLINC, in different treatment conditions. We found that ox-LDL treatment enhanced the expression level of GAPLINC (Figures 1 E and 1 F), indicating that ox-LDL treatment can induce GAPLINC expression in HAECs. Furthermore, the different concentrations and time of the ox-LDL treatments seem to have an effect on the expression of miR-183-5p and downstream molecule PDCD4 (Figures 1 G–J). The relationship between lncRNA GAPLINC and the two specific molecules awaits further study.
Figure 1
Expression of GAPLINC was detected in ox-LDL-induced cells. A, B – CCK-8 assay assessing cell proliferation ability in different times and concentrations of ox-LDL treatment conditions. C, D – Flow cytometry analysis of cell apoptosis rate in different times and concentrations of ox-LDL treatment conditions. *P < 0.05, **p < 0.01, ***p < 0.001. E, F – Expression level of GAPLINC quantified by qPCR in different times and concentrations of ox-LDL treatment conditions. G, H – Relative expression level of miR-183-5p quantified by qPCR. I, J – Relative expression level of PDCD4 quantified by qPCR. Mean ± SD were presented, and experiments were done in triplicate. *P < 0.05, **p < 0.01, ***p < 0.001
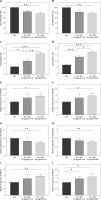
GAPLINC modulated cell apoptosis under ox-LDL treatment
Next, we investigated the role of GAPLINC in HAECs under different ox-LDL treatment conditions. Using our previously established GAPLINC overexpression and shRNA knock-down system [15], we found that overexpressing of GAPLINC significantly inhibited cell proliferation, while silencing of GAPLINC significantly restored cell proliferation ability (Figure 2 A). There are two possible mechanisms that may affect cell proliferation: cell apoptosis and cell cycle alteration. To further investigate the detailed roles of GAPLINC in cell proliferation inhibition, we examined these two possibilities. The results of the experiment (Figure 1) showed that ox-LDL treatment induced cell apoptosis. To test the first hypothesis, we performed a cell apoptosis assay. Flow cytometry analysis demonstrated that overexpression of GAPLINC significantly induced cell apoptosis while silencing of GAPLINC significantly reduced cell apoptosis (Figure 2 B). Consistently, the cell apoptosis results were confirmed by using TUNEL assay (Figure 2 C). We used flow cytometry to investigate the proportion of cells in each cell cycle after propidium iodide (PI) staining to further investigate GAPLINC’s effect on cell cycle progression. GAPLINC overexpression increased the frequency of cells in the G2/M phase and decreased the G0/G1 phase, as shown in Figure 2 D. In the GAPLINC silencing condition, the opposite effects were observed. Surprisingly, we discovered that GAPLINC overexpression significantly increased the proportion of cells in the S phase compared to silencing. Our findings showed that overexpressing GAPLINC in HAECs cells could stop the cell cycle in the S and G2/M phases, inhibiting cell proliferation further.
Figure 2
Function of GAPLINC in ox-LDL-induced cells. The cells were treated with normal conditions, GAPLINC siRNA, GAPLINC overexpression, ox-LDL treatment, ox-LDL treatment with GAPLINC condition, ox-LDL treatment with GAPLINC siRNA and ox-LDL treatment with GAPLINC overexpression, respectively. A – CCK-8 assay assessing cell proliferation ability. B – Flow cytometry analysis of cell apoptosis rate. *P < 0.05, **p < 0.01, ***p < 0.001. C – Cell apoptosis rate accessed by TUNEL assay. *P < 0.05, **p < 0.01, ***p < 0.001. D – Cell cycle profiles were examined by flow cytometry. Mean ± SD were presented, and experiments were done in triplicate. *P < 0.05, **p < 0.01, ***p < 0.001
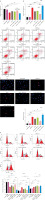
PDCD4 and miR-183-5p are downstream targets of GAPLINC
We and other studies have found that lncRNA GAPLINC overexpression down-regulated miR-211 and up-regulated Bcl2 protein expression in HUVEC cells, resulting in increased cell proliferation [15, 21]. In contrast, GAPLINC lncRNA also has been shown to interact with miR-183-5p directly and up-regulate downstream molecules PDCD4, resulting in cell apoptosis (Figure 3 A). Therefore, further investigation of downstream molecules of GAPLINC in HAECs could elucidate the mechanisms of atherosclerosis in our current system. We next measured the mRNA expression levels of PDCD4, Bcl2, miR-183-5p and miR-211. We observed that overexpressing GAPLINC significantly downregulated the expression of both miR-183-5p and miR-211, suggesting the interaction between lncRNA with miRNAs. Importantly, we also found that overexpression of GAPLINC significantly enhanced the expression of PDCD4 while Bcl2 expression level was reduced significantly and the opposite effects were observed when silencing GAPLINC (Figure 3 B). Moreover, the correlation analysis indicated that the expression of PDCD4 was negatively correlated with miR-183-5p, suggesting that miR-183-5p could regulate PDCD4 expression (Figure 3 C). Western blot analysis showed the same results at the protein level (Figure 3 D).
Figure 3
GAPLINC interaction with miR-183-5p and PDCD4. The cells were treated with normal conditions, GAPLINC siRNA, GAPLINC overexpression, ox-LDL treatment, ox-LDL treatment with GAPLINC condition, ox-LDL treatment with GAPLINC siRNA and ox-LDL treatment with GAPLINC overexpression, respectively. A – A hypothesized model of GAPLINC downstream interaction molecules. B – Relative mRNA expression level of PDCD4, Bcl2, miR-183-5p and miR-211*P < 0.05, **p < 0.01, ***p < 0.001. C – Spearman correlation between miR-183-5p and PDCD4 was assessed. D – Relative protein expression levels of PDCD4 and Bcl2. Mean ± SD were presented, and experiments were done in triplicate. *P < 0.05, **p < 0.01, ***p < 0.001
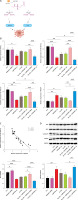
GAPLINC directly interacted with miR183-5p and modulated cell apoptosis
While the interaction between miR-183-5p and PDCD4 has been predicted computationally and validated functionally in gastric cells, the interaction between GAPLINC and miR-183-5p is less well confirmed. It is unknown whether miR-183-5p regulates PDCD4 effectively in HAECs cells. GAPLINC’s relationship to the two molecules remained unknown. To investigate this, we constructed reporter plasmids by cloning the wild type or mutant GAPLINC 3′UTR region downstream of the firefly luciferase reporter. The constructs were then co-transfected with miR-183-5p into HAECs for the luciferase reporter assay. When WT GAPLINC and miR-183-5p mimics were co-transfected into HAECs, the luciferase signal was significantly decreased (Figure 4 A) compared to the mutant vector or negative control. Additionally, we constructed PDCD4 reporter plasmids with wild type or mutant 3′UTR regions. In comparison to the mutant vector or the negative control, co-transfection of PDCD4 and miR-183-5p mimics resulted in a significant decrease in the luciferase signal in HAECs (Figure 4 A), implying that PDCD4 and miR-183-5p interact directly. As a result, these findings show that a high concentration of ox-LDL causes the expression of GAPLINC, which acts as a decoy to sequester miR-183-5p and prevent it from binding to its target PDCD4. The role of miR-183-5p in HAECs treated with ox-LDL was also confirmed. We discovered that overexpressing miR-183-5p significantly inhibited cell death and that silencing miR-183-5p had the opposite effect, which is consistent with what we found in the GAPLINC condition, confirming that miR-183-5p is a downstream target for GAPLINC (Figure 4 C).
Figure 4
GAPLINC directly interacted with miR183-5p and modulated cell apoptosis. A – Luciferase reporter assay was performed to validate the direct target association of GAPLINC, miR-183 and PDCD4. The cells were treated with normal conditions, miR183-5p siRNA, miR183-5p overexpression, ox-LDL treatment, ox-LDL treatment with miR183-5p condition, ox-LDL treatment with miR183-5p siRNA and ox-LDL treatment with miR183-5p overexpression, respectively. B – Relative mRNA expression level of PDCD4 and miR-183-5p. C – CCK-8 assay assessing cell proliferation ability. *P < 0.05, **p < 0.01, ***p < 0.001. D – Flow cytometry analysis of cell apoptosis rate. E – Cell apoptosis rate accessed by TUNEL assay. *P < 0.05, **p < 0.01, ***p < 0.001. F – Cell cycle profiles were examined by flow cytometry. Grouped as G0/G1, G2/M and S stage; Mean ± SD were presented, and experiments were done in triplicate. *P < 0.05, **p < 0.01, ***p < 0.001
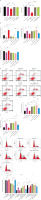
Additionally, PDCD4 mRNA levels were inversely correlated with miR-183 expression (Figure 4 B), implying that decreased PDCD4 expression could be a result of increased miR-183 expression. Additionally, we validated the effects on cell apoptosis and proliferation by measuring viability, apoptosis, and cell cycle progression (Figures 4 D–F).
Silencing of GAPLINC rescued the repressive effects of miR-183-5p
We performed a rescue experiment to further confirm that the effects we observed were specifically due to GAPLINC and miR-183-5p. Our data suggested that silencing of GAPLINC increased cell proliferation and decreased cell death. Further detailed cell cycle analysis indicated that the cell cycle was arrested at the G2/M stage. Of note, silencing of GAPLINC and miR-183-5p partially rescued the cell proliferation ability in the cells (Figures 5 A–D). Taken together, the observations showed that GAPLINC acts as a decoy to sequester miR-183-5p to prevent it from binding to target PDCD4, resulting in cell apoptosis (Figure 5 E).
Figure 5
Rescue experiments on GAPLINC. A – Relative expression level of GAPLINC, PDCD4 and miR-183-5p. Cells were treated with normal conditions, 50 μg of ox-LDL, 50 μg of ox-LDL-NC si-GAPLINC, si-miR-183-5p or both siRNA. B – CCK-8 assay assessing cell proliferation ability. *P < 0.05, **p < 0.01, ***p < 0.001. C – Cell apoptosis rate accessed by TUNEL assay and flow cytometry analysis of cell apoptosis rate. D – Cell cycle profiles were examined by flow cytometry. E – Graphic model of mechanisms of GAPLINC. Mean ± SD were presented, and experiments were done in triplicate. *P < 0.05, **p < 0.01, ***p < 0.001
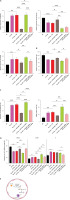
Discussion
Atherosclerosis is a chronic, multifactorial disease characterized by lipid deposition in the vessel wall, which initiates an inflammatory and proliferation cascade involving smooth muscle, endothelial, and immune cells [22]. Accumulating evidence indicates that lncRNAs play critical roles in various stages of plaque development and may offer novel diagnostic and therapeutic strategies for reducing the burden of atherosclerosis, the exact mechanism of which remains unknown [11]. The purpose of this study was to determine the effect of lncRNA GAPLINC on atherosclerosis. Additionally, we demonstrated that lncRNA GAPLINC acts as a decoy for miR-183-5p, preventing it from binding to its target PDCD4, resulting in apoptosis in HAECs.
Oxidized low-density lipoprotein (ox-LDL) plays a critical role in atherosclerosis pathogenesis, in part by promoting endothelial cell dysfunction, proliferation, apoptosis, and necrosis [23, 24]. Numerous studies have demonstrated that oxidized LDLs are cytotoxic towards vascular cells and induce apoptosis, resulting in the release of lipids and lysosomal enzymes into the subendothelial space, thereby accelerating the progression of the atherosclerotic plaque and the formation of the necrotic core and even contributing to the formation of lipid-laden macrophages [2, 22, 24–26]. Numerous cellular processes, such as the remodeling of chromatin, transcription, and post-transcriptional processing, have been linked to lncRNAs [11, 27]. The role of GAPLINC in HAECs and the potential role of lncRNA in atherosclerosis were investigated in this study. Previous research has shown that the RNA GAPLINC plays a role in angiogenesis in HUVEC by targeting miR-211 and then increasing Bcl2 expression, resulting in cell proliferation, implying that GAPLINC can act as a microRNA decoy [15, 18]. This lncRNA is predicted to target a different miR-183-5p, which has been shown to suppress apoptosis and promote proliferation in cancer cells by targeting PDCD4 molecules. According to current evidence, the lncRNA GAPLINC targets either miR-211 or miR-183-5p, which have opposing effects. The expression of miR-183 and of PDCD4 were found to have a negative correlation, according to our findings. GAPLINC may target via miR-183-5p, according to previous research, while interaction with miR-211 in HUVECs may be different than in current HAECs. GAPLINC would most likely target a variety of downstream genes, allowing it to perform a variety of biological functions on different cells.
We discovered that overexpressing GAPLINC at a higher ox-LDL concentration induced cell apoptosis, whereas silencing GAPLINC significantly reduced cell apoptosis. Additionally, GAPLINC inhibited cell proliferation by arresting the cell cycle at the S and G2/M phases. To validate the direct target association of GAPLINC, miR-183, and PDCD4, a luciferase reporter assay was used. There are primarily two conflicting roles for miR-183 that have been investigated previously in various cancer cells. Numerous studies indicate that miR-183 promotes cell growth and motility, while others indicate that miR-183 inhibits cell invasion and migration. MiR-183-5p overexpression suppressed the expression of the PDCD4 gene and expedited cell progression in the G0/G1 phase transition, while also increasing cell proliferation, according to the findings of the current study. Invasion and migration have not been studied in HAECs because of the unique characteristics of these cells. PDCD4 has been identified as a gene whose expression increases following the induction of apoptosis and as a target of miR-183, which binds to the 3′UTR of PDCD4. Over-expression of miR-183 has been shown to inhibit expression of the PDCD4 protein, according to research [18, 28]. Our current study demonstrated that the GAPLINC/miR-183-5p/PDCD4 pathway is a key pathway for regulating endothelial cell death; however, when we silenced both GAPLINC and miR-183-5p, we observed that the cell apoptosis rate was still slightly upregulated compared with the GAPLINC silence only condition in both assays, implying that other molecules or pathways might be involved in this regulation. Therefore, whether other microRNAs or molecules can be regulated by GAPLINC remains unclear and needs to be further explored, e.g. by unbiased RNAseq techniques or GAPLINC pull-down experiments.
Moreover, we also attempted to study an animal model using APOE-fed C57BL/6J mice, and endovascular aortic veins were sampled. One of the limitations is that the lncRNA GAPLINC sequence has not been identified in mice, and in this study we used human GAPLINC primers to test the expression levels, and we did not detect any GAPLINC expression in mice. However, we observed that in mice expressing miR-183-5p the expression level was decreased in APOE-fed mice, and those mice showed significant cell apoptosis of aortic endothelial cells. Meanwhile, in the published articles [29–31], many pioneers have investigated similar mechanisms and obtained significant results. If the GAPLINC gene sequence in mice is clear, we believe that this pathway can be validated.
In conclusion, the current study demonstrated that the GAPLINC/miR-183-5p/PDCD4 pathway was essential to regulate endothelial cell death. Together, these results suggest that GAPLINC may be a promising target to treat atherosclerotic vascular disease.