Introduction
Pediatric intussusception is one of the most common causes of bowel obstruction in the pediatric population. Affected children have one section of the intestine sliding into the adjacent section [1, 2]. Intestinal ischemia-reperfusion injury (I/R) can occur during pediatric intussusception [3, 4], and any delay in diagnosis or treatment can lead to loss of intestinal viability that requires bowel resection [5, 6].
In clinical practice, the diagnosis of pediatric intussusception is mainly based on a combination of clinical symptoms and examinations from ultrasound (US) imaging, computed tomography (CT), or air enema [7]. Early detection of pediatric intussusception and associated intestinal I/R can improve prognosis and treatment efficacy [8, 9]. Until now, there has been no clinical biomarker for early diagnosis of pediatric intussusception and associated intestinal I/R.
Transfer ribonucleic acid (tRNA)-derived fragments (tRFs), a novel type of small non-coding RNA originating from tRNAs [10, 11], participate in many pathological processes [12]. These fragments can be released and detected in the peripheral circulation. Levels of tRFs can fluctuate in response to stimuli. Circulating levels of tRFs might be useful as surrogate measurements of disease progression [13, 14]. Mishima et al. reported that in pathological stress damage, such as ischemic reperfusion, toxic injury, and irradiation, the levels of circulating tRNA derivatives showed a rapid increase [15]. However, no previous study has investigated the role of tRFs and tRNA-derived stress-induced RNA (tiRNAs) in pediatric intussusception and associated intestinal I/R. In our previous studies, we found I/R in both our animal models and our pediatric intussusception patients. We also found that I/R is associated with upregulation of p38MAPK in intussusception [3, 4, 6]. In the current study, we examined whether a gene expression profile could be used for early detection of pediatric intussusception. Specifically, we conducted genome-wide transcriptional profiling using blood-derived tRFs and tiRNAs followed by real-time polymerase chain reaction (PCR) verification in children with intussusception.
Material and methods
Material
We performed a cross-sectional study. The study protocol was approved by the Ethics Committee of our hospital and complied with Helsinki Declaration. Written informed consent was obtained from their parents. Demographic and clinical data were recorded for each study participant between August 1, 2019 and December 31, 2019 at our hospital. The diagnosis of intussusception was based on combined clinical symptom with the US examination. Once the diagnosis was made, air enema was performed as the treatment method.
RNA extraction
Peripheral blood samples were drawn immediately after the final diagnosis by air enema before reduction of intussusception and mononuclear cells were isolated and then stored at –80°C for RNA extraction. We isolated total RNA using TRIzol Reagent (Invitrogen, Carlsbad, California, US) per the manufacturer’s protocol.
Library preparation and tRF/tiRNA sequencing and data analysis
We used agarose electrophoresis to check the integrality of total RNA samples, and then quantified the samples using a NanoDrop ND-1000 Spectrophotometer (Thermo Scientific, Thermo Fisher Scientific, Waltham, Massachusetts, USA). In order to remove RNA modifications that interfere with small-RNA sequencing (RNA-seq) library construction, total RNA samples were first pretreated as follows: 3-aminoacyl (charged) deacylation to 3-OH for 3-adaptor ligation; 3-cP (2,3-cyclic phosphate) removal to 3-OH for 3-adaptor ligation; 5-OH (hydroxyl group) phosphorylation to 5-P for 5-adaptor ligation; and m1A and m3C demethylation for efficient reverse transcription. We took pretreated total RNA from each sample for tRF and tiRNA sequencing (tRF-seq, tiRNA-seq) library preparation. The procedures included: (1) 3-adapter ligation; (2) 5-adapter ligation; (3) complementary deoxyribonucleic acid (cDNA) synthesis; (4) PCR amplification; and (5) size selection of ∼134–160-bp PCR amplified fragments (corresponding to ∼14–40-nt small RNAs). The completed libraries were quantified using an Agilent 2100 Bioanalyzer (Agilent Technologies, Inc., Santa Clara, California, US). We mixed the libraries in equal amounts according to the quantification results and used them for further sequencing.
We denatured DNA fragments in well-mixed libraries with 0.1 M NaOH to generate single-stranded DNA molecules and then loaded them onto the reagent cartridge at a concentration of 1.8 pM. The sequencing was performed on a NextSeq system using a NextSeq 500/550 V2 kit (#FC-404-2005; Illumina, Inc., San Diego, California, USA) per the manufacturer’s instructions. We performed 50 cycles of sequencing.
We analyzed images and performed base calling using Solexa Pipeline v1.8 software (Off-Line Basecaller software v1.8; Illumina). Sequencing quality was examined using FastQC software [16]. We aligned trimmed reads (pass Illumina quality filter, trimmed 5,3-adaptor bases by cut adapt [17]), allowing for only one mismatch to mature tRNA sequences. Then, we aligned the reads that did not map using Bowtie software [18], allowing for only one mismatch to precursor tRNA sequences. The remaining reads were aligned using miRDeep2 software [19], allowing for only one mismatch to microRNA (miRNA) reference sequences. We were then able to calculate the expression profiling of tRFs, tiRNA, and miRNA based on counts of reads mapped. Differentially expressed tRFs, tiRNAs and miRNAs were screened based on the count value using the edgeR package in R software [20]. We performed principal component analysis (PCA), correlation analysis, and hierarchical clustering to create pie, Venn, scatter, and volcano plots in an R or Perl environment for statistical computing and graphics of the expressed tRFs and tiRNAs. The functions and pathways of almost all tRFs were classified according to Gene Ontology (GO) assignment and the Kyoto Encyclopedia of Genes and Genomes (KEGG) database. We used Cytoscape software (version 3.8.0, https://cytoscape.org/) to predict the network of the top 100 potential targets of tRFs and tiRNAs.
Real-time quantitative reverse transcription polymerase chain reaction assay
Using quantitative reverse-transcription polymerase chain reaction (qRT-PCR), we selected and quantified the upregulated expression of tRF-Leu-TAA-006, tRF-Gln-TTG-033, and tRF-Lys-TTT-028 in both study groups. We performed qPCR using a SYBR Green PCR Kit (Applied Biosystems, Foster City, California, USA) per the manufacturer’s instructions. We normalized gene expression to β-actin messenger RNA (mRNA). Relative expression of the gene transcript was calculated using the 2–ΔΔCt method.
Statistical analysis
We used SPSS software (version 20.0, IBM, Armonk, New York, USA) for statistical analysis. Continuous data are presented as mean ± standard deviation (SD). Inter-group comparisons were performed by the rank sum test. Receiver operator characteristic (ROC) curve analysis was conducted and the area under the ROC curve (AUC) was calculated to evaluate the diagnostic accuracy of selected genes in pediatric intussusception. P < 0.05 was considered statistically significant.
Results
Demographic and clinical data
A total of 20 pediatric-intussusception patients and 20 healthy controls were included in the study. Patients’ demographic and clinical data are summarized in Table I. There was no statistically significant difference between the children with intussusception and healthy controls in terms of age (months, 30.97 ±34.94 vs. 35.21 ±40.78, p = 0.511) and gender (male, 10 vs. 12, p = 0.781).
Table I
Demographic and clinical data of pediatric intussusception
Differentially expressed serum tRF and tiRNA levels in patients and controls
In this study, we detected 732 tRFs and tiRNAs, 1705 miRNAs, 52 differentially expressed miRNAs, and 34 differentially expressed tRFs and tiRNAs between the two groups (Figures 1 A, B). We found nine types of tRFs and tiRNAs in both groups, including tRF-1, tRF-2, tRF-3a, tRF-3b, tRF-5a, tRF-5b, tRF-5c, tiRNA-3, and tiRNA-5 (Figures 2 A–C). As shown in Figures 1 A and B, compared with controls, patients had 33 upregulated miRNAs, 24 upregulated tRFs and tiRNAs, 19 downregulated miRNAs, and 10 downregulated tRFs and tiRNAs (fold change, cutoff 1.5; p-value, cutoff 0.05).
qRT-PCR validation
Next, in order to validate differential expression, we used qPCR to measure three strong candidates, tRF-Leu-TAA-006, tRF-Gln-TTG-033, and tRF-Lys-TTT-028, in the 20 patient samples and 20 control samples. The results showed that the expression trend of these three tRFs was consistent with the sequencing results; the difference was statistically significant (Figure 3).
Predictive value of specific tRFs and tiRNAs as diagnostic biomarkers of pediatric intussusception
The ROC curves for differentiating between pediatric-intussusception patients and healthy controls were based on expression levels of tRF-Leu-TAA-006, tRF-Gln-TTG-033 and tRF-Lys-TTT-028. tRFs and tiRNAs with AUC > 0.5 and p < 0.05 were considered appropriate biomarkers. The results showed that the AUCs of tRF-Leu-TAA-006, tRF-Gln-TTG-033 and tRF-Lys-TTT-028 were 0.984 (95% confidence interval (CI): 0.952–1.000; p < 0.05), 0.970 (95% CI: 0.933–1.000; p < 0.05) and 0.837 (95% CI: 0.722–0.951; p < 0.05), respectively (Figure 4).
Prediction of potential targets for differentially expressed tRFs and tiRNAs and functional analysis of target genes
We performed GO and KEGG pathway analyses to analyze target genes in order to explore the potential functions and mechanisms of tRFs and tiRNAs in pediatric intussusception. Classification of tRF and tiRNA target genes was based on the cellular component (CC), molecular function (MF), and biological process (BP) categories. Gene Ontology annotations demonstrated that the target genes of tRF-Gln-TTG-033 were abundant in the function of material metabolism, such as the nucleic acid metabolic process, heterocycle metabolic process, organonitrogen compound metabolic process, nitrogen compound metabolic process, and organic-substance metabolic process (Figure 5 A). In addition, the target genes of tRF-Gln-TTG-033 were primarily found in the organelle, intracellular compartment, and cell part, while the MFs of its products mainly included binding, protein binding, and transferase activity (Figure 5 B). After mapping the targeted genes of tRF-Gln-TTG-033 in the KEGG database, we found that these genes participated in the “AMPK signaling pathway”, “FoxO signaling pathway”, “Cellular senescence” and “Relaxin signaling pathway”, which were associated with I/R (Figure 5 C).
Figure 5
Gene Ontology (GO) and pathway analysis of tRF-Gln-TTG-033. Target genes were predicted by Miranda algorithms and TargetScan miRNA prediction programs
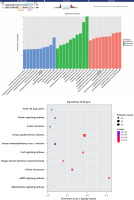
Regarding tRF-Leu-TAA-006, BP-based terms revealed that tRF target genes were related to the cellular protein modification process and the protein modification process (Figure 6 A). In addition, the target genes of tRF-Leu-TAA-006 were primarily found in the intracellular membrane-bound organelle, intracellular compartment, and cell part; and the MFs of its products mainly included binding, protein binding and ion binding (Figure 6 B). After mapping the targeted genes of tRF-Leu-TAA-006 in the KEGG database, we found that these genes participated in the “RAS pathway” and “Protein procession”, which were associated with I/R (Figure 6 C).
Figure 6
Gene Ontology (GO) and pathway analysis of tRF-Leu-TAA-006. Target genes were predicted by Miranda algorithms and TargetScan miRNA prediction programs
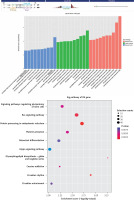
For tRF-Lys-TTT-028, target genes played a role in lipid metabolic processes, including the nucleic acid metabolic process, macromolecule metabolic process, cellular macromolecule metabolic process, and RNA metabolic process (Figure 7 A). In addition, these genes were primarily found in the intracellular membrane-bound organelle, intracellular compartment, and cell part, and the MFs of its products mainly included DNA binding, heterocyclic compound binding, and organic cyclic-compound binding (Figure 7 B). After mapping the targeted genes of tRF-Lys-TTT-028 in the KEGG database, we found that these genes participated in “Signaling pathways regulating pluripotency of stem cells” and “Taurine and hypotaurine metabolism”, which were associated with I/R (Figure 7 C).
tRF-target gene interaction network analysis
Having revealed the correlation between tRFs and pediatric intussusception, we next explored interactions between target genes predicted by our biological analysis to better understand the intrinsic mechanisms of tRFs. Using the Miranda (http://www.microrna.org/) and RNAhybrid (M. Rehmsmeier, 2004) tools, we found the top 100 potential targets of tRF-Leu-TAA-006, tRF-Gln-TTG-033, and tRF-Lys-TTT-028, as shown respectively in Figures 8 A–C.
Discussion
Intussusception is one of the most common causes of bowel obstruction in children. I/R in intussusception can result in mucosal erosion and hemorrhagic ulceration, as well as necrosis of the bowel, lung injury, and multiple-organ dysfunction syndrome [21]. Oxidative stress can play a crucial role in I/R in pediatric intussusception [22]. Some research shows that early intervention has a protective effect in I/R [23, 24]. So early detection of intestinal I/R in intussusception can improve prognosis and reduce complications in the intestines and extraintestinal organs. Although many efforts have been made to identify biomarkers of pediatric intussusception and associated I/R, no definite biomarkers are currently available in clinical practice.
Non-coding RNAs (ncRNAs) can be released to circulate in the peripheral blood. Some studies have shown that tRFs are related to translation, gene expression, and the cellular stress response. The mechanisms underlying tRFs regulate some human diseases, such as viral infections, cancers, pathological stress damage, neurological diseases and metabolic diseases [12, 15]. Circulating levels of tRFs could be useful as biomarkers of some diseases and surrogate measures of disease progression [13, 14], indicating their potential use in diagnosis and assessment of I/R in pediatric intussusception. In our previous studies, we found I/R in both our animal models and our pediatric intussusception patients. The diagnosis of existing intussusception cannot provide the clues for precise reflection of how tRFs are related to translation, gene expression, and the cellular stress response. Therefore, we decided to test the expression of tRFs in serum from patients and further focus on biological analysis to verify whether circulating tRFs could serve as potential biomarkers in pediatric intussusception and associated I/R.
In the present study, we first performed high-throughput sequencing (HTS) to detect the expression profiles of tRFs in pediatric intussusception patients. To validate differential expression in the sequencing data, we selected three dysregulated tRFs for qRT-PCR review to verify the authenticity of the profiles. We detected the most significantly upregulated tRFs—tRF-Leu-TAA-006, tRF-Gln-TTG-033, and tRF-Lys-TTT-028 – in serum samples from patients. Our qRT-PCR results demonstrated that all three tRFs were significantly upregulated in patients compared with controls. Via GO analysis, we learned that the targeted genes of these differentially expressed tRFs were involved in metabolism, protein modification, binding, transferase activity, protein binding, DNA binding, heterocyclic compound binding, and organic cyclic-compound binding. In mapping all targeted genes to the KEGG database, we noted that they participated in the following: “AMPK signaling pathway”, “FoxO signaling pathway”, “Cellular senescence”, “Relaxin signaling pathway”, “RAS pathway”, “Protein procession”, “Signaling pathways regulating pluripotency of stem cells” and “Taurine and hypotaurine metabolism”. This is consistent with findings in the literature that these signaling pathways play important roles in I/R [25–32]. However, the involvement of these dysregulated tRFs and their target genes in I/R in the pediatric intussusception process via these signaling pathways requires further studies.
To apply the expression values of serum tRFs and tiRNAs for clinical diagnosis, we analyzed the ROC curves. We found that serum tRF-Leu-TAA-006, tRF-Gln-TTG-033, and tRF-Lys-TTT-028 were significantly upregulated in pediatric intussusception children compared with controls. The results showed that AUCs of tRF-Leu-TAA-006, tRF-Gln-TTG-033, and tRF-Lys-TTT-028 were 0.984, 0.970, and 0.837, respectively (Figure 5). These findings suggested that serum levels of these three tRFs might be involved in the development of pediatric intussusception and could become new biomarkers for pediatric intussusception. The present study found higher levels of tRFs, the targeted genes of these differentially expressed tRFs and the associated pathway. Thus, it may provide clues for precise decision making for treatment of intussusception associated with I/R.
There are some limitations of the present study. First, the sample size was relatively small. Second, only three tRFs identified in microarray experiments were validated via qRT-PCR. Future studies should be conducted with larger sample sizes and in-depth verification of more candidate genes. Finally, the potential mechanisms of tRF-Leu-TAA-006, tRF-Gln-TTG-033, and tRF-Lys-TTT-028 in pediatric intussusception and how the changes of tRFs targeting pathways affect the progression of the disease still require further studies.
In conclusion, the present study provided an overall analysis of tRFs and tiRNAs in pediatric intussusception and indicated that tRF-Leu-TAA-006, tRF-Gln-TTG-033, and tRF-Lys-TTT-028 could play important roles in pediatric intussusception. The results of further biological analysis suggested that these three tRFs could serve as novel serological biomarkers with significant accuracy in diagnosing pediatric intussusception. We believe that our research could offer clues for further research into the mechanism of tRFs in pediatric intussusception.