Introduction
Osteosarcoma is the most common primary malignancy of the bone, occurring predominantly in adolescents and children. Data from 1973–2004 suggested that the incidence of osteosarcoma was approximately 4.4 cases per 1 million people who were aged 24 years or younger [1]. Osteosarcoma was treated primarily with surgical resection (amputation or limb-salvage surgery). With the adjuvant treatment with pre-op and/or post-op chemotherapy, the survival of osteosarcoma has been remarkably improved over the past several decades [2, 3]. One study showed that the 5-year survival in osteosarcoma patients under 15 years was increased from 40% to 76%, and in adolescent patients from 56% to 66% between 1975 and 2010 [4]. Nevertheless, the existing regimens including combinational use of high dose methotrexate, doxorubicin, cisplatin, epirubicin, ifosfamide, cyclophosphamide, etoposide, gemcitabine and topotecan have been limited by the significant systemic side effects such as hepatic and neural toxicity. Further, a subset of patients responded poorly to the established chemotherapy or relapsed after therapy [5]. Their prognosis has shown little or no improvement over the past 20 years. As such, considerable efforts have been actively spent to develop novel agents that can be used alone, or in combination with current standards of care, for the management of osteosarcoma.
Resveratrol (Res) is a polyphenolic phytoalexin natural compound obtained from various fruits and red wine. Initially recognized for its anti-inflammatory, anti-angiogenic and anti-oxidative effects, Res has been widely studied for the anti-tumor activities in various cancer models [6–9] since the first report in a mouse skin cancer model [10]. Significant evidence has suggested that Res has chemotherapeutic or chemopreventive effects on several cancer types [11–13]. For example, Res suppressed the growth and metastasis of SW480 human colon cancer cells via the induction of microRNA 663 [14]. A study by Zulueta et al. additionally showed that Res altered intracellular signaling necessary for cell growth arrest and apoptosis in gastric carcinoma [15].
Given the existing evidence, it is imperative to investigate the efficacy of Res in osteosarcoma.
There have been a number of relevant studies available from the public databases. Initially, Zou et al. screened 14 botanical extracts and found that Res had significant anti-osteosarcoma activities in blocking cell proliferation and activating apoptosis [16]. These events were associated with the inhibition of canonical Wnt/β-catenin signaling. Further, Res inhibited HIF-1α protein expression and hypoxia-enhanced proliferation, invasion, and epithelial to mesenchymal transition in osteosarcoma [17]. Li et al. found that Res induced apoptosis in four osteosarcoma cell lines: HOS, Saos-2, U2-OS and MG-63 [18]. The authors postulated that the effects of Res were mediated through silent information regulator 2 (Sirt1), given that a similar effect was elicited by a Sirt1 activator, isonicotinamide, and that resveratrol was enhanced by nutrition restriction by L-asparaginase. An study by Liu et al. likewise investigated the cell apoptosis in MG-63 cells by resveratrol, wherein the authors observed that Res could inhibit growth and induce apoptosis [19]. Preliminary observational data suggested the molecular mechanism related to the modulation of survivin and p21. Finally, Res had an inhibitory effect on vascular endothelial growth factor expression and U2-OS cell proliferation [20]. Thus, it appeared generally accepted that Res induces cytotoxicity in osteosarcoma, yet possibly through diverse molecular mechanisms and intracellular processes. Significant further work is also needed to attract the clinical use of Res in oncology, as opposed to the dietary use from its well-known cardiovascular protective activity.
In this study, we used the MG-63 osteosarcoma cell culture model and assessed the chemotherapeutic effect of Res. In line with earlier publications, our results suggested that Res blocks cell proliferation, migration, and invasion, and activates apoptotic cell death in osteosarcoma. Notably, we instead found that Res potentially down-regulates nuclear factor κB (NF-κB) and Akt intracellular signaling cascades. Res, when combined with an NF-κB inhibitor, pyrrolidine dithiocarbamate (PDTC), results in synergistic and pronounced growth inhibition of osteosarcoma. Together, our in vitro preclinical study is among the first to report the synergistic action of Res and PDTC, and supports the translation of Res (alone or in conjunction with NF-κB inhibition) to the clinical management of patients with osteosarcoma.
Material and methods
Materials
Resveratrol and pyrrolidine dithiocarbamate were purchased from Sigma-Aldrich (St Louis, MO, USA). Res was dissolved in dimethyl sulfoxide (DMSO) and PDTC was dissolved in phosphate-buffered saline (PBS). Both reagents were stored at –80°C. Tetrazolium dye MTT and sodium pyruvate were acquired from Aladdin (Shanghai, China). Matrigel basement membrane matrix was purchased from BD Biosciences (NJ, USA).
Cell culture
The human osteosarcoma cell line MG-63 was obtained from iCell (Shanghai, China). MG-63 cells were maintained in the Minimum Essential Media (MEM) culture medium (Gibco, MA, USA), supplemented with 10% fetal bovine serum (FBS, Gibco, MA, USA), 100 kU/l penicillin and 100 mg/l chloramphenicol (Gibco, MA, USA) and 0.11 g/l sodium pyruvate at 37°C with 5% carbon dioxide (CO2). Cells are routinely passaged with 0.25% trypsin digestion. Changes in cell morphology and growth were monitored with an inverted optical microscope.
MTT assay
MG-63 cell proliferation was determined by the tetrazolium dye 3-(4,5-dimethyl-2-thiazyl)-2,5-diphenyl-2H-tetrazolium bromide (MTT) assay. Briefly, 5 × 103 MG-63 cells (100 μl) in the exponential phase of growth were plated in 96-well culture plates. After 24 h, cells were divided into three groups: group one was treated with Res (0, 25, 50, 100 or 200 μM), group two was treated with PDTC (0, 3, 10, 30, 50 or 100 μM), and group three was treated with the combination of Res (fixed at 100 μM) and PDTC (0, 3, 10, 30, 50 or 100 μM). Cells were continued to culture for 24 or 48 h. Then, 100 μg MTT (20 μl) of reagent was added to each well. After 4 h of incubation, 150 μl of DMSO was added to each well to dissolve the formed crystals under oscillation. Finally, enzyme-linked immunosorbent assay (ELISA) was performed to determine cell proliferation at 570 nm. Groups one and two were calculated with the following formula: rate of inhibition (%) = (1 – absorbance in experimental group/absorbance in control group) × 100%. For group three, Jin’s method was used to determine whether the two drugs had synergistic effects [21]. This Q value model was also known as the probability addition method, a widely used algorithm modified from Bürgi’s equation suitable for studying combinational use of traditional or herbal medicine. The equation was as follows: Q = EAB/(EA + EB – EA × EB). EAB represented growth inhibition of the combination group, whereas EA or EB represented the inhibition respectively achieved in A (100 μM Res) or B (3, 30, 50, or 100 μM PDTC). A Q value between 0.85 and 1.15 indicated a simple addition, whereas > 1.15 indicated a synergistic effect and < 0.85 an antagonistic effect.
Wound healing assay
MG-63 cells were seeded in 6-well plates until reaching 80% confluency. Three straight wounds were introduced to each well with 200 μl pipette tips. Detached cells during the procedure were rinsed off with phosphate-buffered saline (PBS). The adherent cells were treated with 100 μM Res for 24, 48 or 72 h at 37°C with 5% CO2. Microphotographs were taken at 0, 24, 48 and 72 h to record cell migration. Image-Pro Plus 6.0 software (Cybernetics, Princeton, NJ) was employed to quantify the cell migration and calculate the migration index.
Transwell migration assay
MG-63 cells untreated or pretreated with Res (25, 50, 100 or 200 μM) for 48 h were collected by 0.25% trypsin digestion and centrifugation. Cells were re-suspended in culture medium at 5 × 105/ml. Transwell permeable supports were purchased from Corning (NJ, USA). The upper compartments were coated with 50 μl of Matrigel (1 g/l) at 37°C for 1 h to form artificial basement membranes. Next, 200 μl of MG-63 cells were plated in the upper chambers, and 600 μl of medium supplemented with 20% FBS was added to the lower compartments. Cells were cultured for 48 h. The permeable filters were fixed with 4% paraformaldehyde. The cells from the upper surface were scraped off. The cells at the lower surface were stained with 0.05% crystal violet and counted with microscopy. The following equation was used to determine cell migration and invasion: (1 – invasive cells in the experimental group/invasive cells in the control group) × 100%.
TUNEL assay
MG-63 cells were plated on coverslips in 24-well plates at a density of 1 × 105/ml and cultured for 24 h. Res was added to the culture medium at a final concentration of 0, 25, 50, 100 or 200 μM. After 24 h, apoptosis in MG-63 cells was examined with the TUNEL Apoptosis Detection Kit (FITC) following the manufacturer’s instructions (San Yi Biotechnology, Shanghai, China). Apoptotic cells emitted green fluorescence at 520 ±20 nm, whereas the nuclear counterstain with 4’,6-diamidino-2-phenylindole (DAPI) had blue fluorescence at 460 nm. All microphotographs were taken at 20× magnification with scale bars representing 30 μm.
Western blotting analysis
Western blotting analysis of protein expression followed standard procedures. In brief, total proteins were isolated from MG-63 cells treated with Res (0, 25, 50, 100 or 200 μM) for 48 h. Protein extracts were subjected to sodium dodecyl sulfate (SDS)-polyacrylamide gel electrophoresis (PAGE) and transferred to polyvinyl difluoride (PVDF) membranes via electro-blotting. Proteins were detected with the following antibodies: phosphor (p)-Akt, Akt, Erk, p-Erk, NF-κB and caspase-3 (Cell Signaling Technology, MA, US), glyceraldehyde 3-phosphate dehydrogenase (GAPDH) and mechanistic target of rapamycin (mTOR) (Abcam, MA, USA) and goat anti-rabbit secondary antibody (Invitrogen, CA, USA). Densitometry was performed with Image J (National Institutes of Health) following web-based instructions as follows: http://lukemiller.org/index.php/2010/11/analyzing-gels-and-western-blots-with-image-j/.
Statistical analysis
Data were processed with GraphPad Prism 5.0 (GraphPad Software, CA, USA) for figure plotting and statistical analysis. The normally distributed data were reported as mean ± standard deviation and compared using analysis of variance (ANOVA). All experiments were triplicated. P < 0.05 was considered statistically significant.
Results
Resveratrol inhibits osteosarcoma cell proliferation
Res has been tested for its anti-tumor activities in a variety of in vitro and animal models, including the two recent cell culture studies in osteosarcoma [16, 18]. To validate the results in our cell line model, we first tested the effect of Res on MG-63 osteosarcoma cell proliferation. With the MTT assay, we observed time- and dose-dependent suppression of cell growth. At 24 h, 100 μM and 200 μM Res had statistically significant effects compared to the untreated group (Figure 1 A). The calculated IC50 for 24 h was 333.67 μM. With prolonged exposure for 48 h, we saw the cytotoxic sensitivity extended to as low as 50 μM (Figure 1 B). The calculated IC50 for 48 h was reduced to 253.5 μM. This was consistent with the clinically relevant doses used by other researchers.
Figure 1
Resveratrol (Res) inhibits osteosarcoma MG-63 cell proliferation. A – 24 h of Res treatment at 100 and 200 μM significantly inhibited MG-63 cell proliferation. ***p < 0.001 compared to the untreated control, which was not plotted in the graph. B – At 48 h, as low as 50 μM Res showed significant growth inhibition of osteosarcoma MG-63 cells. ***p < 0.001 compared to the untreated control. All experiments were triplicated with the data expressed as mean ± standard deviation
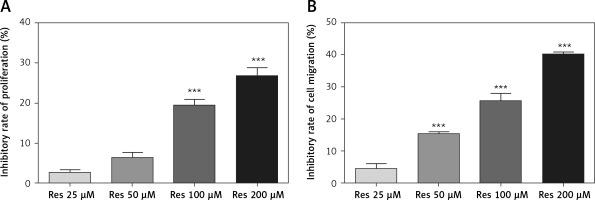
Resveratrol suppresses osteosarcoma cell migration
Cell migration is the key step in tumor progression from primary neoplasm to disseminated disease. We next assessed the efficacy of Res in inhibiting cell migration. We used the wound healing assay, a well-established assay of studying cell polarization, tissue remodeling, cell proliferation and migratory behaviors. Shown in Figure 2 A, MG-63 osteosarcoma cells treated with 100 μM Res displayed a temporarily diminished migratory ability and wound-healing process. This result, when quantified, showed a statistically significant difference from the untreated cells (Figure 2 B).
Figure 2
Res suppresses osteosarcoma MG-63 cell migration. A – Representative microphotographs (20×) of the wound healing assay. MG-63 cells were treated with 100 μM Res for 24, 48 or 72 h. Solid lines represent migratory front edges. B – Quantitation of cell migration in wound healing assay. Compared to the control (ctrl), 100 μM Res treatment for 48 and 72 h showed pronounced inhibition of osteosarcoma cell migration (***p < 0.001). All experiments were triplicated with the data expressed as mean ± standard deviation
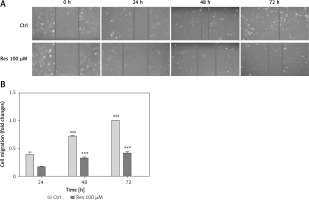
Resveratrol reduces osteosarcoma cell invasion
Resveratrol has been shown to hamper cell migration, with some authors suggesting that the action occurred primarily through inhibiting matrix metalloproteinase in multiple myeloma [22] and regulating Rho GTPases in breast carcinoma [23]. In osteosarcoma, a study by Yang et al. found that Res inhibited tumor migration, invasion and metastasis, possibly through the transcriptional suppression of MMP (matrix metalloproteinase)-2 via miR (microRNA)-328 [24]. With the Transwell assay, we indeed observed dose-dependent inhibition of cell invasion through the artificial basement membrane (Figure 3 A). In comparison to growth inhibition (Figure 1), Res had a higher potency in suppressing cell invasion, with 25 μM showing a significant difference from the untreated cells (Figure 3 B).
Figure 3
Res reduces osteosarcoma MG-63 cell invasion. A – Representative microphotographs (20×) of MG-63 cells in the Transwell assay. Cells were pretreated with 25, 50, 100 or 200 μM Res. B – Quantitation of the cell invasion in Transwell assay. Res pretreatment significantly reduced osteosarcoma cell invasion across the artificial basement membrane (***p < 0.001). All experiments were triplicated with the data expressed as mean ± standard deviation
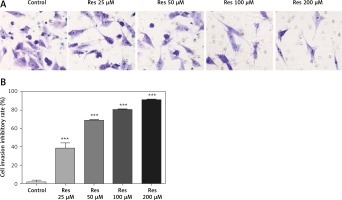
Resveratrol promotes osteosarcoma apoptotic cell death
In earlier work [18], Res was shown to induce apoptosis in osteosarcoma cells with the Annexin V staining method. Annexin V is a calcium-dependent phospholipid-binding protein that has a high affinity for phosphatidylserine, which is translocated from the inner plasma membrane to the cell surface in apoptosis. Here we used the TUNEL assay to detect the endonucleolytic cleavage of chromatin, an event largely characteristic of late apoptosis. Shown in Figure 4, MG-63 cells treated with Res showed dose-dependent accumulation of DNA fragmentation (Figure 4 A). With 24 h of treatment, as low as 25 μM Res was capable of elucidating a strong apoptotic signal (Figure 4 B).
Figure 4
Res promotes MG-63 osteosarcoma apoptotic cell death. A – Representative immunofluorescence microphotographs of the TUNEL assay. MG-63 cells were treated with 25, 50, 100 or 200 μM Res for 24 h. FITC detected the apoptotic cells (circled) and DAPI stained nuclei of all cells. Note the gradual decline in viable cells treated with high concentrations (100 and 200 μM) of Res. B – Quantitation of apoptotic cell death in TUNEL assay. Compared to the control (ctrl), Res significantly promoted cell apoptosis in osteosarcoma MG-63 cells (**p < 0.01, ***p < 0.001). All experiments were triplicated with the data expressed as mean ± standard deviation. Microphotographs were taken at 20× magnification with scale bars representing 30 μm
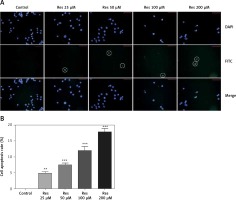
Resveratrol regulates NF-κB and Akt signaling in osteosarcoma
Past studies have suggested that Res suppresses carcinogenesis by modulating intracellular signal transduction pathways. The affected signal network governs multiple crucial processes including cell division, growth, apoptosis, inflammation, angiogenesis, and metastasis. In particular to osteosarcoma, such alterations included Wnt/β-catenin signaling and the Sirt1/Sir2 family of NAD-dependent histone deacetylases [16, 18]. As an initial approach to probe the mechanistic basis of Res’s anti-osteosarcoma activity, we subjected the total protein extracts from Res treated MG-63 cells to Western blotting analysis (Figure 5 A). With caspase-3 antibody, we observed dose-dependent cleavage of the full-length caspase-3 and accumulation of the active form (quantified via densitometry, Figure 5 B). This was consistent with the observation that Res activated the canonical apoptotic pathway seen in Figure 4. Further, Res treatment resulted in dose-dependent suppression of NF-κB, a key transcription complex of immune response and carcinogenesis. When used for 48 h, as low as 25 μM had a significant impact on NF-κB, when normalized to GAPDH and compared to the control (Figure 5 C). Akt is a serine/threonine kinase that is involved in diverse biological responses including inhibition of apoptosis and stimulation of cell proliferation. Although the total Akt level responded poorly to Res treatment, the phosphor (p)-Akt showed a dose-dependent reduction, suggesting that its phosphorylation and activation were suppressed by Res (Figures 5 D, E). We also tested mechanistic target of rapamycin (mTOR), another serine/threonine kinase that has an integral role in cell growth, motility, survival, and transcription. We found that Res treatment had a neglectable effect on mTOR expression, only evident at the highest concentration, 200 μM (Figure 5 F). The Erk and p-Erk we tested showed a discordant response to Res treatment. At 100 and 200 μM Res, total Erk showed an increase in protein expression (Figure 5 G), whereas the p-Erk was modestly suppressed (Figure 5 H). Indeed, the effect was more pronounced when p-Erk was normalized with total Erk (results not shown). If further verified, Erk might be another downstream target of Res’s pharmacological action.
Figure 5
Res regulates Akt and NF-κB signaling in MG-63 osteosarcoma cells. A – Representative Western blotting analysis of various proteins affected by Res in MG-63 osteosarcoma cells. MG-63 was treated with 25 to 200 μM Res for 48 h. Glyceraldehyde 3-phosphate dehydrogenase (GAPDH) was used as loading control. B–H – Densitometric quantitation (Image J) of relative expression of cleaved caspase-3 (B), NF-κB (C), phosphor (p)-Akt (D), Akt (E), mechanistic target of rapamycin (mTOR) (F), Erk (G) and p-Erk (H). **p < 0.01, ***p < 0.001. All experiments were triplicated with the data expressed as mean ± standard deviation
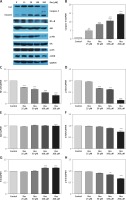
Synergistic effect of Res and NF-κB inhibitor pyrrolidine dithiocarbamate
In our results, Res treatment resulted in dose-related suppression of NF-κB, a protein complex with a recognized role in inflammation and cancer. Chemical inhibitors of NF-κB are readily available, and many efforts have been invested to bring experimental drugs to clinical use. Our experiments suggested that NF-κB could be a downstream target of Res. Thus, the combination regimen of Res with an NF-κB inhibitor should achieve synergistic cytotoxicity. Among the list of NF-κB inhibitors, PDTC is well established for its role as an NF-κB inhibitor and antioxidant with pro-apoptotic and anti-proliferative activities across various cancer models. However, its cytotoxic profile in osteosarcoma has not been established. In this experiment, we treated MG-63 cells with increased doses of PDTC for 24 or 48 h. At 24 h, 50 and 100 μM of PDTC showed significant inhibition of osteosarcoma proliferation in the MTT assay (Figure 6 A, IC50: 429.3 μM). Extending the treatment to 48 h, we found that as low as 30 μM of PDTC was effective in reducing cell survival (IC50: 411.4 μM). Next, we combined Res and PDTC. For Res, we used a fixed concentration of 100 μM, a concentration tolerated by the cells for subsequent survival analysis. For PDTC, in addition to 30 to 100 μM, we also included 3 and 10 μM. We reasoned that since PDTC is a direct NF-κB inhibitor, it may sensitize osteosarcoma to Res treatment even at below-the-threshold concentrations. Indeed, we observed strong synergistic activity between the two drugs at all combinational doses tested for 24 or 48 h (Figures 6 C, D and Table I).
Table I
Synergistic effects between resveratrol (Res) and pyrrolidine dithiocarbamate (PDTC) in inhibiting MG-63 osteosarcoma proliferation
Figure 6
Synergistic effect of Res and NF-κB inhibitor pyrrolidine dithiocarbamate (PDTC) in suppressing MG- 63 osteosarcoma proliferation. A – At 24 h, 50 and 100 μM of PDTC significantly reduced MG-63 cell proliferation. The calculated IC50 for PDTC at 24 h was 429.3 μM. The calculated IC50 for PDTC at 48 h was 411.4 μM. B – At 48 h, as low as 30 μM of PDTC had a significant impact on MG-63 cell survival. For A and B: *p < 0.05, **p < 0.01. All experiments were triplicated with the data expressed as mean ± standard deviation. Combinational use of Res and PDTC in MG-63 osteosarcoma cells at 24 h (C) and 48 h (D). All experiments were triplicated with the data expressed as mean ± standard deviation
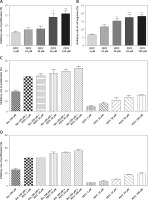
Discussion
Osteosarcoma is an aggressive primary bone tumor, leading to cancer mortality in a matter of months without clinical management. Even though there has been significant progress in the past decades, many patients failed to achieve clinically desired therapeutic outcomes. Hence, novel and more effective chemotherapies are urgently needed.
Res has been of interest lately to some researchers for its anti-tumor effects in osteosarcoma. Driven by this shared interest, we proposed the current study investigating the molecular basis of the cytotoxicity of Res in MG-63 osteosarcoma cells. We found that Res inhibited proliferation and migration, and induced apoptosis in a concentration- and/or time-dependent manner. This finding is corroborated by earlier studies [16–20, 24]. We also found that Res down-regulates the NF-κB intracellular signaling cascade. Further evidence was collected from the combination study; Res, when combined with the NF-κB inhibitor PDTC, resulted in synergistic and more pronounced growth inhibition of osteosarcoma.
NF-κB is a ubiquitously expressed gene capable of regulating a wide spectrum of target genes. The NF-κB signaling pathway is likely aberrantly activated in many human solid tumors, including colorectal, cervical, prostate, pancreatic, breast and thyroid cancer [25–30]. Further, NF-κB signaling also appears to intertwine with other signaling cascades such as Akt signaling. As a matter of fact, the Akt pathway was reported to converge with NF-κB signaling at IκBα kinase (a substrate of Akt) even though these two cascades were initially considered separate [31]. Another biological link between NF-κB and PI3-kinase/AKT has also been suggested in the anti-apoptotic modulation of PEL cells [32].
In a number of past studies, Res showed significant effects on the regulation of NF-κB signaling. Kundu et al. noted that Res inhibited NF-κB activation through suppressing the phosphorylation and subsequent degradation of IκBα in TPA-treated mouse skin [33]. Res also acted upon NF-κB by inhibiting p65 and IκBβ kinase [34]. Hence, based on the existing evidence and our present findings, we reason that NF-κB and Akt signaling pathways may be altered by Res to mediate the cytotoxicity in MG-63 cells.
Combination chemotherapies are often more efficacious in anti-tumor activity compared to the single-agent approach in cancer treatment. Encouraged by Res-induced regulation of NF-κB, we examined the synergistic effect of Res and PDTC. The results indicated that combination treatment gave greater inhibition of tumor proliferation. Res has been tested in a list of combinatorial strategies (pharmaceutical or non-pharmaceutical) in preclinical models. For example, Joseph et al. suggested that Res altered the apoptotic signaling pathways when combined with caloric restriction [35]. An in vivo study investigated the combination of Res with immunotherapy in mouse neuroblastoma, where the authors noted that the combination therapy led to a significant increase in animal survival and lessened cancer metastasis [7].
Nonetheless, there are several areas of interest that worth further investigation. For one, in the current study, Res inhibited the proliferation of MG-63 cells only at concentrations exceeding 50 μM. The bioactivity of Res at lower doses remains unclear or less promising. A past study suggested that low-dose Res paradoxically enhanced cell survival preceding the later inhibition of cell survival [36]. In contrast, Chin and colleagues reported that low-concentration Res (0.1 μM) consistently induced a decrease in cancer cell proliferation [37]. Second, the effect of Res on the Erk/p-Erk pathway opens another discussion. Our finding of inhibition of Erk phosphorylation by Res was supported by Lee et al., who investigated the antioxidant effect of Res on the fibrosarcoma cell line HT1080 [38]. On the other hand, the study by Niles et al. stated that Res activated phosphorylation of Erk in the human melanoma cells A 375 [6]. These discrepancies will have to be resolved by further studies.
In conclusion, our preclinical study demonstrates that Res inhibits cell proliferation, migration, and invasion, and activates apoptosis in the osteosarcoma MG-63 cell line model. This is likely mediated through the inhibition of NF-κB, given that the combination of Res and NF-κB inhibition results in synergistic growth inhibition. Our findings thus support the translation of Res to the clinical evaluation of patients with osteosarcoma.