Red blood cell (RBC) transfusion (RBCT) is one of the most frequently performed hospital procedures. Transfusion decision making (TDM) represents a common challenge to critical care physicians [1]. On one hand RBCT may be associated with complications such as acute lung injury, circulatory overload, transmission of pathogen, immunosuppression, and increased mortality [1]. In this context RBCT should be viewed as a treatment of last resort in anemic critically ill patients. Anemia management should instead be focused on causal anemia treatment and minimization of patient blood loss, both important elements of patient blood management [2]. On the other hand, anemia in critically ill patients may lead to, among other consequences, prolonged weaning from the respirator, acute kidney injury, myocardial ischemia [3], the need for RBCT.
Numerous scientific societies recommend that TDM should be based on hemoglobin (Hb) concentration and clinical symptoms. In critically ill patients clinical symptoms of anemia may be masked by sedation or neurologic impairment. Tolerance of anemia in a particular patient depends on the fine balance between global O2 delivery (DO2) and consumption (VO2). Physiological parameters that reflect the DO2-VO2 balance cover but are not limited to: arterial-venous oxygen difference (A-V O2diff), O2 extraction ratio (O2ER), mixed venous O2 saturation (SvO2), central venous O2 saturation (ScvO2), lactate concentration. The A-V O2diff is calculated as the difference between arterial [CaO2 = SaO2 × Hb × 1.34 + (PaO2 × 0.0031)] and central venous [CcvO2 = ScvO2 × Hb × 1.34 + (PcvO2 × 0.0031)] O2 content. A-V O2diff was shown to be a moderate independent predictor of 90-day mortality in transfused and non-transfused patients [4]. As a surrogate of SvO2 in the aforementioned equation ScvO2 may be used, but it does not take into account O2 consumption of the myocardium. In pathophysiologic states where myocardial O2 consumption cannot be ignored, there is no agreement between ScvO2 and SvO2 [5]. Apart from states of increased myocardial O2 consumption, ScvO2 is considered a good surrogate of DO2-VO2 balance when Hb is fully saturated with O2. Another surrogate of DO2-VO2 balance that has been used is lactate, although its interpretation may be particularly difficult in septic patients [6]. Pharmacological agents commonly used in the critically ill (e.g. β2-adrenergic receptor agonists, epinephrine) may increase lactate concentration. Regional tissue ischemia may lead to elevated lactate. Clearance of lactate may be impaired in liver dysfunction. Hyperglycemia stimulates glucose conversion into lactate [7]. Due to numerous limitations, lactate concentration should be used with caution in these clinical situations. Moreover, our recent retrospective analysis showed that RBCT did not normalize mildly increased lactate in non-bleeding severely anemic critically ill patients and this probably was not due to factors potentially increasing lactate in this patient population. Therefore we concluded that lactate may have a limited role as a physiologic RBCT trigger in this specific cohort of patients [8]. It should be bear in mind that these parameters are only surrogates of DO2-VO2 balance and should be interpreted with caution, especially ScvO2 and lactate; therefore TDM should incorporate as many surrogates as possible.
We present our original physiologic approach to RBCT in Figure 1.
Figure 1
Physiologic red blood cell transfusion algorithm in non-bleeding critically ill patients
A-V O2diff – arterial-venous oxygen difference, CI – cardiac index, CO – cardiac output, CRT – capillary refill time, DO2 – global oxygen delivery, Hb – hemoglobin concentration, IVCd – inferior vena cava diameter, PaO2 – arterial partial pressure of oxygen, P(v-a)CO2 – venousarterial carbon dioxide difference, RBC – red blood cell, SaO2 – arterial oxygen saturation, ScvO2 – central venous oxygen saturation, SpO2 – peripheral oxygen saturation, SvO2 – mixed venous oxygen saturation, VO2 – global oxygen consumption *Consider other causes of elevated lactate: sepsis, hyperglycemia, liver dysfunction, epinephrine, β2-adrenergic agonists, regional tissue ischemia.
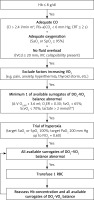
Only if the former statement in the algorithm is confirmed can the next statement be considered. The starting point in our algorithm is Hb concentration determination. Restrictive compared to liberal RBCT strategy (transfusion at Hb ≤ 7 g/dl vs. ≤ 9 g/dl) is safe and potentially reduces in-hospital mortality in critically ill adults [9]. However, patients with acute coronary syndrome or chronic cardiac disease may benefit from a higher transfusion trigger [10]. Therefore we implement the algorithm at Hb ≤ 8 g/dl. As RBCT at restrictive triggers may not improve DO2 in some patients and may even be deleterious, we work through the algorithm. Even elderly patients with chronic heart failure may tolerate very low Hb concentrations [11]. According to the equation DO2 = CO × [(1.34 × Hb × SaO2) + (0.0031 × PaO2)], apart from Hb DO2 depends on other factors, therefore before assessing surrogates of the DO2-VO2 balance, normal cardiac output (CO) (CI ≥ 2.4 l/min m2), normal SaO2 (≥ 95%), and no volume overload should be assured. For CO measurement there are various methods in use: transpulmonary thermodilution, arterial pressure-based cardiac output monitoring (calibrated and uncalibrated), etc. As a surrogate of adequate CO one may use venous-arterial CO2 difference (Pv-aCO2), which should be < 6 mm Hg [12]. When advanced hemodynamic monitoring is not available (e.g. no central line in the upper body), satisfactory organ perfusion may be assured by checking the capillary refill time (CRT) [13]. Fluid overload should be excluded as it leads to hemodilution and simple fluid de-resuscitation may increase Hb concentration to satisfactory levels. Exclusion of fluid overload is difficult, ultrasound techniques might be helpful here – inferior vena cava diameter (IVCd) and its collapsibility. Factors increasing VO2 should be excluded. Next, surrogates of DO2-VO2 balance should be determined. To improve the objectivity of the results obtained we advise to use all surrogates available in a particular patient, although not all will be available in all patients. Four out of five mentioned surrogates require cannulation of internal jugular or subclavian vein for sampling central venous blood. If the pulmonary artery is already cannulated it should be used for sampling mixed venous blood in order to obtain the most accurate results. If at least one of the available surrogates is abnormal, a trial of moderate hyperoxia should follow. The aim of hyperoxia is to fully saturate Hb with O2 and increase the amount of O2 transported in plasma. Increase in SaO2/SpO2 from 95 to 100% in patients with a Hb concentration of 8 g/dl should increase the amount of O2 carried by Hb by 0.54 ml/dl. An increase in PaO2 from 80 to 200 mm Hg should increase the amount of O2 transported in plasma by 0.37 ml/dl. These extra amounts of O2 (0.91 ml/dl in total), at this particular Hb concentration, constitute a 9% increase in arterial oxygen content. These extra amounts of O2 are not negligible as they amount to almost as much O2 as carried by 1 g of Hb in dl at SaO2 95% (1.27 ml). The goal for PaO2 is 200 mm Hg and this value is derived from perioperative bleeding guidelines, where PaO2 > 200 mm Hg is considered excessive hyperoxia [14]. We warn against using high fractions of inspired O2 (FiO2 > 0.60) to aim for PaO2 200 mm Hg, leading to lung injury especially if used for a prolonged time. Satisfactory PaO2 can be reached by measures other than increasing FiO2: continuous positive airway pressure, positive end-expiratory pressure, increasing inspiration-expiration ratio, prone position, etc. A trial of moderate hyperoxia should relatively quickly improve surrogates which depend directly on SaO2 and O2 transported in plasma (A-V O2diff, O2ER, SvO2 and ScvO2). Changes in lactate require longer time and it is suggested that reassessment should be performed after 1–2 h [15]. Following the hyperoxia trial, all available surrogates should be reassessed. If all available surrogates are abnormal we advise transfusion of 1 unit of RBC. According to best practices we support a 1 unit transfusion policy. Following RBCT Hb concentration and all available surrogates should be reassessed in a time-sensitive manner and the decision whether to transfuse another RBC unit made.