Introduction
Thyrotropin (TSH, thyroid-stimulating hormone) is a heterodimeric glycoprotein hormone, composed of α and β subunits. TSH level in peripheral blood is an important predictor and it is generally the first step screening test for thyroid functions, but even in the normal range it may be affected by some non-thyroidal situations in the body [1] and may affect thyroid tissue even after thyroid operations [2, 3]. The α subunit is common in TSH, FSH and LH, whereas β-subunits are different in these pituitary hormones. TSH stimulates the thyrocytes via the G-protein coupled receptor and causes production of thyroid hormones [4]. Regulation of TSH synthesis occurs via negative feedback of thyroid hormones and stimulation by thyrotropin-releasing hormone (TRH) from the hypothalamus [5]. Adenohypophysis has 2 main parts in humans: the pars distalis (PD) and pars tuberalis (PT). Thyrotroph cells of PD and PT differ morphologically; PT thyrotrophs have fewer granules, but more Golgi, whereas PD thyrotrophs have more dense secretory granules on electron microscopy. In the late 1990s, another important point was revealed; studies showed that PT thyrotrophs do not have TRH receptors and TSH secretion in PT is independent from TRH in these cells [6]. Instead of TRH receptors, PT thyrotroph cells have the MT-1 melatonin receptor and TSH secretion from PT cells is regulated by melatonin released by photoperiodic stimuli [7, 8]. Melatonin suppresses TSH-β chain expression via MT-1 receptors in PT-thyrotrophs [9, 10]. In a study with C57BL mice that have a defect in the melatonin gene causing an intensified effect of external melatonin, TSH secretion was decreased by melatonin injection in PT thyrotrophs, whereas it had no effect in PD cells and TSH decreased in the periphery after melatonin injection [11]. It was an interesting finding that although peripheral TSH was decreased, serum T4 level was not affected, indicating lower bioactivity of TSH from PT.
Thyroid-stimulating hormone glycoforms
Intrapituitary and circulating TSH consists of several isoforms with heterogenous carbohydrate branching with variable terminal residues that cause differences in ability to interact with specific regions of the TSH receptor. Glycosylation is an important step to regulate TSH bio- and immuno-activity.
Primary hypothyroidism, thyroid hormone resistance and TRH administration increase oligosaccharide addition, resulting in increased bioactivity of TSH [12, 13]. Central hypothyroidism [14], TSH-producing pituitary adenomas and euthyroid sick syndrome also cause different TSH-glycosylation patterns [15]. Changes in sulphation and sialylation of oligosaccharide residues could also be seen [16, 17]. Decreased sialylation caused increased bioactivity [18]. Highly sialylated TSH has prolonged half-life in serum but has low intrinsic bioactivity.
Studies of Ikegami et al. showed that glycosylation of PD-TSH and PT-TSH differs. PD-TSH has biantennary glycans whereas PT-TSH has multi-branched, sialylated N-glycans. Tissue-specific expression of glycosyltransferases causes these differences in oligosaccharide chains [11].
Photoperiodism
The pineal gland (epiphysis), which was thought to be the ”seat of the soul” by Descartes centuries ago, is important in regulation of the circadian rhythm in mammals. Knowledge of light coming from the eyes passes through the suprachiasmatic nucleus and arrives at the pineal gland to regulate melatonin synthesis. Melatonin is secreted in darkness and it is the signal for the ”length of the night” to the whole brain [19]. All creatures make necessary changes in their physiology and behaviours (hibernation, breeding, etc) to adapt to climate changes and organisms mostly take ”length of the day” into consideration as knowledge of seasonal changes, instead of temperature, because the temperature may not always reflect the season correctly.
Small mammals and birds breed during spring and summer months and they are known as ”long day breeders”. Their pregnancy or incubation periods are short and their babies are born within spring or early summer.
Bigger mammals such as sheep and goats breed in autumn. They have a longer gestation and give birth in summer, so they are ”short day breeders”.
In all vertebrates reproductive activity is regulated by the hypothalamo-hypophyseal-gonad axis. In birds, photoperiodism changes gonadal volumes up to 100 times, whereas in mammals this is not more than up to a few times [20].
When melatonin-proficient CBA mice were raised under short-day (SD) conditions, expression of TSH-β was suppressed in the PT, but not in the PD. When T3 was injected daily into these mice to suppress PD-TSH, PD-TSH-β mRNA and serum TSH decreased. When these mice were transferred to long day (LD) conditions to induce production of PT-TSH, PT-TSH-β and serum TSH increased but serum T4 did not change [11]. Therefore PT-TSH was shown to have low bioactivity at the periphery. To analyse differences in glycosylations of these two molecules, the same team performed western blotting. PD-TSH (37 kDa) and PT-TSH (40 kDa) had different molecular weights (MW), but when deglycosylated their MW were same. PT-TSH had multi-branched sialylated N-glycans, and it was found that these two molecules had a difference in glycosylation. PT-TSH was shown to have low bioactivity, by producing a macro-molecule by binding with IgG2b and albumin [21, 22]. Studies on Chinese hamster ovary cell (CHO) lines showed that these two TSHs caused the same cAMP accumulation, but TSHs from SD-breeder (higher PD-TSH) and LD-breeder (higher PT-TSH) rats had different activities towards these cell lines, and also macro-TSH had very low activity in humans and rats [21, 23].
PT-TSH has longer bioactivity due to decreased hepatic clearance because of sialylation, albumin covalent, probable transglutamin covalent binding [24] (Figure 1).
Figure 1
PT-TSH and PD-TSH and actions. PD-TSH stimulates the thyroid gland and is regulated by TRH and negative feedback of thyroid hormones, in accordance with classical endocrinology knowledge. PT-TSH does not have a significant peripheral role but mainly acts on the hypothalamus, causing seasonal changes. The action of PT-TSH needs melatonin in mammals, but PT-TSH secretion is directly activated by light affecting deep brain receptors in birds without a melatonin signal (adapted and modified from [11])
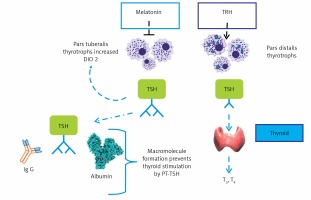
Thyroid hormones, seasons and reproduction
In most birds, mammals and fish, thyroid hormones are known to be related to seasonal reproductive functions. In some species, thyroidectomy prevents seasonal testicular growth and L-thyroxine replacement mimics the long-day effect by suppressing PD-TSH but does not affect PT-TSH [25–27]. The ideal animal model for photoperiodism studies is the Japanese quail (Coturnix japonica), which has rapid responses. Local illumination of the mediobasal hypothalamus (MBH) induces testicular growth and lesions of this region block the photoperiodic response of LH secretion and gonadal development in these animals [28–31]. Because in birds, for photoperiodism melatonin is not needed, birds have deep brain photoreceptors and PT-TSH is directly stimulated; therefore the pineal gland is not involved, as has been known for many years [32, 33]. For example, local illumination of the septal region of the telencephalon or the MBH using radioluminous-painted beads caused testicular growth in quail, suggesting the existence of deep brain photoreceptors in these regions [34, 35]. Interestingly, mammalian neural tissue opsin 5 may be a deep brain photoreceptive molecule in the quail brain to detect light [36].
Yoshimura et al. reported induction of the deiodinase-2 (DIO-2) gene and suppression of the deiodinase-3 (DIO-3) gene by LD stimuli, in ependymal cells lining ventrolateral walls of the third ventricle within MBH, by using differential subtractive hybridization analysis [37, 38]. Among these cells, tanycytes respond to PT-TSH. DIO-2 enzyme increases active thyroid hormone triiodothyronine (T3) production, whereas DIO-3 encodes thyroid hormone-inactivating enzyme that metabolizes T4 to inactive reverse T3 (rT3) and 3,3’-diiodothyronine (T2). T3 concentration within the MBH is about 10-fold higher under LD conditions. Intracerebroventricular T3 infusion in SD conditions induced testicular development while infusion of the DIO-2 inhibitor iopanoic acid in LD conditions attenuated testicular development [37]. This photoperiodic regulation of DIO-2 and DIO-3 has also been observed in some other avian species [39–41] and in some mammals [42–46]. Activation of thyroid hormone within the MBH decodes the LD information to the brain. Therefore, daily T3 subcutaneous injections induce testicular development and chronic replacement of T3 in the hypothalamus prevents the onset of testicular regression in LD-breeding Siberian hamsters. In contrast, in the SD breeders, LD-induced DIO-2 appears to convert T4 to T3 to terminate the breeding season. In addition, the LD stimulus induces the expression of DIO-2, and T4 administration terminates the breeding season via a decrease in serum LH [47, 48] (Figure 2).
Figure 2
Deiodinases. Deiodinases in brain tissue regulate active thyroid hormone levels and related hypothalamic effects. DIO-2 enzyme increases T3 production and intracerebroventricular T3 induces testicular development in LD-breeder animals. Photoperiodic switching of DIO-2 to DIO-3 precedes gonadotropin changes in mammals and birds (see [38])
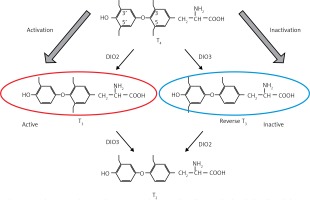
In quail, the photoperiodic switching of DIO-2 and DIO-3 precedes photoperiodic induction of gonadotropin release by nearly 4 h [49]. Before this switching, the gene encoding the TSH-β subunit is induced. In the ependymal cells TSH receptors are expressed. Several rhodopsin families are involved in deep-brain-sensing of light in avians, including rhodopsin, melanopsin, neuropsin, and vertebrate ancient opsin [50]. However, in mammals eyes are the only photoreceptor organ. Therefore removal of eyes abolishes the photoperiodic response [19]. Light information from the eye is transferred to the suprachiasmatic nucleus (circardian pacemaker) and then to the pineal gland. In all mammals, pinealectomy abolishes seasonal responses and melatonin administration restores them.
Melatonin has melatonin-1 (MT1) and melatonin-2 (MT2) receptors. But these melatonin receptors are not expressed in the ependymal cells where DIO-2 and DIO-3 are expressed. The MT1 receptor is expressed in the thyrotroph cells of the pars tuberalis [7]. Therefore PT-TSH probably mediates the influence of melatonin on DIO-2/DIO-3 switching in mammals. As expected, clear photoperiodic regulation of TSH-β, DIO-2, and DIO-3 was observed in the melatonin-producing CBA mice strain, while such responses were not observed in the melatonin-deficient C57BL strain [9]. In addition, daily intraperitoneal melatonin injections mimicked the effect of SD conditions on the expression of these genes.
Nearly two decades ago thyroid hormones were known to be lipophilic and to traverse cellular membranes by passive diffusion. In fact in mammals, some members of the organic anion transporting polypeptide (Oatp) family are involved in transportation. Oatp1c1 has been shown to be a highly specific transporter of T4 [51]. Another hormone transporter, monocarboxylate transporter 8 (MCT 8), has been found in cells for T4 transport, including cells of mediobasal hypothalamus ependymal cells of hamsters, and its regulation depends on SD conditions, without the need for T4 [51–54].
Intracerebral T3 and gonadotropin interaction
Thyroid hormone receptors are expressed in the median eminence and the ultrastructure of the median eminence was examined via electron microscopy to understand their target site. Dynamic morphological changes were observed between gonadotropin releasing hormone (GnRH) nerve terminals and glial endfeet in the median eminence [55]. In SD conditions GnRH nerve terminals are encased by the endfeet of glial processes and do not contact the basal lamina, while many GnRH terminals are in close proximity to the basal lamina under LD conditions. It has been proposed that the nerve terminals of hypothalamic neurons are required to contact the pericapillary space directly for the secretion of the hypothalamic neurohormone from the hypothalamus into the portal capillary [56]. Morphological changes between the GnRH nerve terminals and endfeet of glial processes are observed in SD quail treated with T3 to stimulate testicular growth and gonadotropin-inhibitory hormone (GnIH) may interfere with this process [57]. Therefore these morphological changes appear to regulate or modulate the seasonal GnRH secretion from the median eminence. It is also interesting to note that the seasonal plasticity within the GnRH system is reported in ewes [58].
Other non-thyroidal photoperiodic effects of thyroid hormone
SD conditions increase the peripheral leukocyte count in hamsters while supressing T3-related RFamide-related peptide-3 mRNA expression, stimulating splenic IL-1β mRNA expression and decreasing splenic TNF-α mRNA expression [59]. Studies in gonadectomized animals show that neuroimmune connections that underlie photoperiodic immune system and disease-related behaviours (anorexia, weight loss, moulting, decreased nest-forming behaviour) are related to pineal gland-gonadal hormones and T3 [60, 61]. Photoperiodic DIO-3 transcription regulation affects T3 stimulation in lymphoid cells and regulates seasonal immune system adaptations [62]. Also food intake and appetite-related mechanisms need local T3-paraventricular nucleus and arcuate nucleus relationships.
Importance of endocrine mechanisms in photoregulation for future
The photoperiodic signalling pathway could be a potential target in domestication, because seasonal breeding is a rate-limiting factor in animal procreation. TSH receptor (TSHR) could be a domestication locus in chicken [63]. The genes involved in the photoperiodic signalling pathway could be good targets for domestication and conversion of LD animals to SD animals or vice versa. Therefore, the mechanism that differs in LD and SD breeders with the same T3 stimulation also need to be elucidated with further studies.
For human beings, the importance of these TSH-related mechanisms could be important for understanding the effects of thyroid-related drugs in fetal brain, melatonin-related effects in space studies, neurological mechanisms in thyroid hormone resistance syndromes and possible use in in vitro fertilisation technology and human reproduction. In human life there is no preferred seasonal breeding period, but effects of thyroid hormone especially on developing fetal brain and possible benefits of knowing the effects of different TSHs on different cells for improving fertilisation could be studied in future. There are still no data or studies on the clinical importance and application of these TSH-related findings to humans.
Although photoperiodic adaptations are more evident in other mammals, presence of such cascades in humans is an interesting topic and recently important reviews were published about the role of hypothalamic and pituitary circuits in seasonal rhythms, related to the stress response and immunity and even colour perception in mammals [64–66]. The pars tuberalis seems to have an important role in seasonal adaptation, hormonal and metabolic responses and immunity via tuberalins and various molecules interacting with other brain areas and systems [65]. This review tries to bring together the available knowledge about the role of the pars tuberalis in photoperiodism and the role of the presence of two different TSHs in that interaction. It is interesting to reveal the molecular economy of nature with two different actions of the same molecule on different sites, behind clinical practice and classically known TSH action. Tissue-specific glycosylation could change the effect of a well-known molecule and may prevent or change some signals in vivo.