Introduction
Five decades ago, Fredrickson, Levy, and Lees (FLL) characterized clinical dyslipidemia phenotypes qualitatively using electrophoresis and ultracentrifugation [1]. They separated disorders of elevated cholesterol and/or triglycerides into five phenotypes (types I–V), each of which represented a characteristic abnormality: chylomicrons (types I, V), very low-density lipoproteins (VLDL) (types IIb, IV, V), low-density lipoproteins (LDL) (types IIa, IIb), or remnant particles (type III). The World Health Organization (WHO) adopted this schema in 1972 [2].
Although modern lipid diagnosis has largely been simplified to identifying elevations in LDL-cholesterol (LDL-C) as reflected in worldwide guidelines, FLL phenotypes remain of prognostic and therapeutic importance [3–6]. Each phenotype has specific implications for atherosclerotic cardiovascular disease as VLDL and remnant particles are highly atherogenic [7, 8]. Furthermore, these phenotypes are associated with non-cardiovascular morbidity including pancreatitis and xanthomata formation [9]. With the expanding armamentarium of lipid-lowering therapies aimed at specific lipoproteins, identifying the presence of a FLL phenotype may also facilitate personalized, targeted therapy [10–13].
In the era of FLL’s seminal publications, lipid fractionation techniques were limited in their scalability to assess prevalence of these phenotypes in large studies [14]. As a result, the prevalence of FLL phenotypes has been uncertain and clinicians have generally viewed them as rare entitities [15–17]. However, the ongoing epidemics of overweight/obesity status and metabolic syndrome/diabetes may affect the modern prevalence of FLL phenotypes, in particular those associated with an excess of triglyceride-rich lipoproteins [9, 18–20]. In addition, Sniderman et al. recently validated a quantitative approach to diagnose the FLL phenotypes integrating the conventional lipid profile with apolipoprotein B (apoB), a measure standardized by the WHO and readily available by most major laboratories worldwide [21, 22].
We therefore sought to determine the contemporary prevalence [15] of the FLL phenotypes in US adults in two settings: a national sample representative of the general population and a large clinical laboratory database reflecting individuals undergoing lipid testing in clinical practice.
Material and methods
Study populations
We examined fasting participants from the National Health and Nutritional Examination Survey (NHANES) 2011–2012 and 2013–2014 cycles, and fasting patients from the second harvest of the Very Large Database of Lipids (VLDL) study [23, 24]. NHANES is a complex survey, conducted by the National Center for Health Statistics, to provide a representative sample of the civilian, non-institutionalized US population. The process of sample selection and participation in NHANES has been fully described previously [25]. This analysis included 5,272 fasting NHANES participants 18 years and older with complete lipid data (Supplementary Figure S1).
The VLDL study was constructed with de-identified data from the clinical operations of a single laboratory (VAP Diagnostics Lab, Birmingham, AL) [24]. The database includes patients from thousands of clinicians across the US in predominantly primary care settings. The process of de-identification and quality checks in the VLDL study was described elsewhere [24]. This cross-sectional analysis included 128,506 fasting patients 18 years and older from the VLDL study with apoB testing (Supplementary Figure S1). If a patient had more than one lipid test in the database, then we included only the first test in this analysis.
NHANES study protocols were approved by the National Center of Health Statistics institutional review board. Written informed consent was obtained from all participants. VLDL study protocols were reviewed by the Johns Hopkins IRB and deemed exempt due to the use of de-identified data only.
Lipid measurements
Within NHANES, total cholesterol, high-density lipoprotein-cholesterol (HDL-C), and triglycerides (TG) were measured photometrically using oxidation reagents. LDL-C and VLDL-cholesterol (VLDL-C) were calculated and apoB was measured through immunochemical reactions and light spectroscopy [26–28]. The full process of lipid measurement, data checking, and quality inspection within NHANES has been documented in detail elsewhere [26–28].
In the VLDL dataset, Vertical Auto Profile (VAP) methodology (VAP Diagnostics Lab, Inc., Birmingham, AL) was used to directly measure the cholesterol content in LDL, VLDL, HDL, and intermediate-density lipoproteins (IDL) [24]. The VAP method uses single vertical spin density gradient ultracentrifugation to separate lipoprotein fractions in less than 1 h. The cholesterol contents of the eluted lipoprotein fractions were measured using a validated colorimetric assay employing cholesterol oxidase reactions. TG values were directly measured with the Abbott ARCHITECT C-8000 system (Abbott Laboratories, Abbott Park, IL). Yearly quality assessments with VAP and Abbott ARCHITECT-derived values have been described previously [24]. ApoB was directly measured through a WHO standardized immunoassay based on immunoturbidimetry with the Abbott ARCHITECT analyzer and reagent.
Definitions of dyslipidemia
We defined conventional dyslipidemia using cutpoints set forth by the National Cholesterol Education Program’s Adult Treatment Panel III guidelines for elevated LDL-C at two targets: ≥ 160 mg/dl and ≥ 130 mg/dl (Figure 1) [3]. In this study, we used the Sniderman algorithm to define the FLL phenotypes, which uses two values from a standard lipid profile (total cholesterol and TG) in conjunction with apoB levels (Figure 1) [21]. This algorithm was derived from a cohort of 1,771 fasting patients with genetically verified dyslipidemias and has been validated using other cohorts [21, 22].
Figure 1
Dyslipidemia classification schema. A – Fredrickson-Levy-Lees classification for types I–V using the apoB algorithm. B – Conventional criteria classification using only LDL-C at two guideline-recommended cutpoints
CE – cholesterol ester, TG – triglyceride, LDL – low-density lipoprotein, VLDL – very low-density lipoprotein, apoB – apolipoprotein B, LDL-C – low-density lipoprotein-cholesterol.
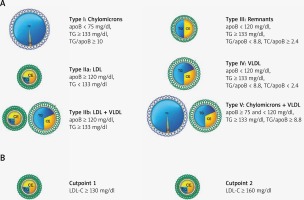
Other variables
In NHANES, demographic and prescription medication information was gathered via in-home interviews and questionnaires [23, 25]. Race categories included: non-Hispanic White, non-Hispanic Black, Mexican American, and other. NHANES defined lipid-lowering therapies as the following: statins, bile acid sequestrants, and cholesterol absorption inhibitors. Body mass index (BMI) was calculated via height and weight measurements, and was categorized as: underweight (< 18.5), normal (≥ 18.5 to < 25), overweight (≥ 25 to < 30), and obese (≥ 30). Diabetes was assessed via measurement of fasting blood glucose and hemoglobin A1c, defined as: fasting glucose ≥ 126 mg/dl, hemoglobin A1c ≥ 6.5%, or self-report of a previous physician diagnosis. In the VLDL study, additional variables included age and sex.
Statistical analysis
In NHANES, we accounted for the complex sampling design and used sampling weights to generate nationally representative estimates in all analyses. We used the Taylor series (linearization) method to calculate standard errors [23, 25]. In the VLDL study, we compared distributions of lipid parameters in participants with available apoB values and those without apoB values. In each study, we examined the prevalence for the overall study populations and stratified by age and sex. Comparisons of prevalence were made using χ2 tests. We calculated medians and interquartile ranges (IQR) for each lipid parameter and by phenotype. We produced kernel density plots for pertinent lipid values. We also used logistic regression in NHANES participants to determine FLL phenotype prevalence by clinical characteristics such as BMI and diabetes marginally adjusted for age, sex, and race/ethnicity. All statistical tests were two-sided with significance at p < 0.05. Statistical analyses were conducted using Stata statistical software version 14.2 (StataCorp, College Station, TX).
Results
Study populations
The 5,272 NHANES participants had a median age of 45 years (IQR: 32–60 years), with 48.4% men (Table I). The majority of NHANES participants were overweight or obese (68.8%). Nearly 1 in 3 NHANES participants were on lipid-lowering therapies, predominately statins. The median age of the 128,506 VLDL study patients was 57 years (IQR: 46–68 years), with 44.8% men, similar to the age (median: 57 years; IQR: 47–67 years) and sex distributions (47.4% men) in VLDL patients without apoB values. Furthermore, lipid distributions and percentiles of total cholesterol, LDL-C, HDL-C, and TG were similar between included VLDL patients compared to patients in the database without apoB values (Supplementary Figure S2).
Table I
Characteristics of NHANES and VLDL study populations
Parameter | NHANESa (n = 5,272) | VLDLb (n = 128,506) |
---|---|---|
Demographics: | ||
Age, median (IQR) [years] | 45 (32–60) | 57 (46–68) |
Men, n (%) | 2,578 (48.4) | 57,221 (44.8) |
Race, n (%): | ||
Non-Hispanic white | 2,173 (66.2) | |
Non-Hispanic black | 1,132 (11.6) | |
Mexican American | 632 (8.6) | |
Other | 1,335 (13.7) | |
BMI category, n (%): | ||
Underweight | 107 (1.7) | |
Normal | 1,610 (29.5) | |
Overweight | 1,639 (32.2) | |
Obese | 1,916 (36.5) | |
Presence of diabetes, n (%) | 906 (12.8) | |
Lipid-lowering therapy, n (%): | 1,058 (33.4) | |
Statin use | 981 (31.4) | |
Lipid characteristics, median (IQR) [mg/dl]: | ||
Total cholesterol | 187 (162–214) | 197 (168–228) |
ApoB | 87 (72–105) | 92 (76–108) |
HDL-C | 51 (43–62) | 52 (43–64) |
VLDL-C | 20 (16–26) | 22 (17–29) |
IDL-Cc | 13 (9–19) | |
LDL-C | 111 (89–136) | 116 (92–144) |
TG | 99 (68–147) | 110 (78–159) |
b Information on race, BMI, co-morbidities and medication use was not available in the VLDL dataset.
c IDL-C was not measured in NHANES. ApoB – apolipoprotein B, HDL-C – high-density lipoprotein-cholesterol, VLDL-C – very low-density lipoprotein-cholesterol, IDL-C – intermediate density lipoprotein-cholesterol, LDL-C – low-density lipoprotein-cholesterol, TG – triglyceride, mg/dl – milligrams per deciliter.
Overall FLL phenotype prevalence
Using conventional criteria, the proportions of participants with LDL-C ≥ 160 mg/dl in NHANES and in the VLDL study were 9.5% and 14.9%, respectively. The corresponding proportions when using LDL-C ≥ 130 mg/dl were 30.3% and 37.2%, respectively. A greater percentage of individuals was categorized as dyslipidemic by the FLL criteria compared to the conventional criteria (p < 0.001). The proportions of individuals with one of the FLL phenotypes were 33.9% in NHANES and 40.3% in the VLDL study. Among NHANES participants with LDL-C < 130 mg/dl or < 160 mg/dl, the proportions of participants with one of the FLL phenotypes were 16.9% and 25.9%, respectively. Corresponding proportions in the VLDL study were 19.6 and 28.8%, respectively.
Individual FLL phenotype prevalence
By individual FLL phenotype, type IV dyslipidemia was the most prevalent in both NHANES and VLDL (prevalence of 20.5% and 24.1%, respectively; Table II), followed by type IIb (prevalence of 8.0% and 10.3%, respectively). Types IIa and III phenotypes were present in 3–4% and 2% of participants in both studies, respectively, while types I and V were rare in both studies.
Table II
Prevalence, % (95% CI) of the FLL phenotypes in NHANES and VLDL study populations
FLL phenotype prevalence by clinical characteristics
The patterns of FLL phenotypes by age and sex groups were similar to that in the overall sample (Table II), although participants 40–74 years of age had a higher overall prevalence of the FLL phenotypes, particularly types IIa, IIb, and IV, compared to other age groups (p < 0.001). Men had greater overall prevalence of the phenotypes compared to women, especially with types IIb and IV (p < 0.001).
Using NHANES data, we further evaluated the marginally adjusted prevalence of FLL phenotypes by race, BMI, and presence of diabetes (Figure 2, Supplementary Table SI). By race, the prevalence of participants with one of the FLL phenotypes was lowest in non-Hispanic Blacks and highest in Mexican Americans (20.5% vs. 42.7%; p < 0.001). The obese group had nearly triple the prevalence, and overweight group had nearly double the prevalence of individuals with any FLL phenotype compared to the normal BMI group (p < 0.001). The diabetes group had nearly double the prevalence of persons with one of the FLL phenotypes compared to participants without diabetes (p < 0.001).
Figure 2
FLL phenotype prevalence by race, BMI, and diabetes
BMI – body mass index (underweight: < 18.5; normal: ≥ 18.5 and < 25; overweight: ≥ 25 and < 30; obese: ≥ 30). Prevalence by race was marginally adjusted for age and sex, while prevalences by BMI and diabetes were marginally adjusted for age, sex, and race.
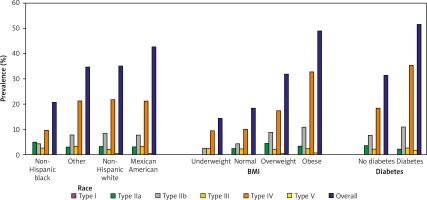
Lipid values
The median levels of total cholesterol, apoB, and TG in NHANES participants without any FLL phenotype were 178, 80, and 79 mg/dl, respectively. The corresponding medians in the VLDL study were 188, 83, and 85 mg/dl, respectively (Supplementary Table SII and Supplementary Figure S3). Individuals with any FLL phenotype in NHANES had median total cholesterol values ranging from 189–253 mg/dl, apoB values of 69–134 mg/dl, and TG values of 171–1,311 mg/dl. The respective ranges in VLDL patients were 149–268 mg/dl, 64–134 mg/dl, and 106–1,143 mg/dl.
The median levels of lipid parameters in each FLL phenotype were similar in both studies, with expected elevations in the pertinent parameters characteristic for each phenotype. For example, in type IV, median VLDL-C values were 30 mg/dl for both NHANES and VLDL populations. In contrast, participants in NHANES with the type IIb phenotype had similar elevations in VLDL-C (36 mg/dl) but also had median LDL-C values of 168 mg/dl. The corresponding medians in Type IV VLDL study patients were 38 and 171 mg/dl, respectively.
Discussion
The key message of our study is that in contemporary samples, the FLL lipoprotein phenotypes of diagnostic, prognostic, and therapeutic relevance appear far more prevalent than historically and clinically appreciated. This is especially true for the type IV phenotype which was present in approximately 20% of the study populations, as well as for rarer phenotypes such as the type III which was found in 2% of participants. A similar high prevalence of the FLL phenotypes was found in a representative sample of the US population and in a large clinical laboratory sample representing predominately primary care patients.
Historical trends
Smaller population studies using electrophoresis and ultracentrifugation previously suggested a low prevalence of certain phenotypes [15–17]. Reported frequencies of the type IIb phenotype ranged from 2–4%, and type III was postulated to occur in < 0.7% of individuals, with prevalence as low as 0.01% depending on the population [15–17, 29, 30]. In our study, the type IIb phenotype was 2–5 times as prevalent, and the type III phenotype 3–4 times as prevalent as previously reported. Both phenotypes are highly atherogenic. The type IIa phenotype, typically associated with familial hypercholesterolemia, also appears to be more common than formerly appreciated. The prevalence of familial hypercholesterolemia is estimated at 1 in 250 (0.4%; or more than 1 million cases in the US) [31, 32]. Yet, the type IIa phenotype had a prevalence nearly 10 times greater (3–4%), likely reflecting non-familial hypercholesterolemia cases. In contrast, the types I and V phenotypes appear to be truly rare, each with a prevalence well below 0.5% as suggested in prior reports [9, 15–17, 33].
The overall high FLL phenotype prevalence, especially types IIb and IV, may be the lipid manifestations of the obesity and diabetes epidemics as these conditions result in hypersecretion of hepatic VLDL [18, 34]. In fact, this was a trend predicted by Fredrickson, Levy, and Lees in their seminal papers [11]. Despite a lack of large-scale databases at the time, the authors postulated that type IV was likely the most prevalent in part due to a large number of secondary causes, particularly diabetes. Nearly 1 in every 3 adult Americans have prediabetes or diabetes and approximately 2 in every 3 are overweight or obese [35]. While smaller cohort studies have suggested a relatively high prevalence of type IV dyslipidemia in specialized populations, our study demonstrates the very high prevalence of this phenotype across age and sex in the general population and its correlation with diabetes and obesity [36].
Our analysis included individuals both on and off lipid-lowering therapies. Lipid-lowering agents may convert patients from one phenotype to another (i.e., from a type IIb to type IV with statin therapy) or to the “normal” category. Given the high prevalence of statin use in the US, our results may underrepresent the true prevalence of the FLL phenotypes, particularly types IIa and IIb phenotypes characterized by excess LDL-C. However, since lipid-lowering drugs have become standard-of-care and part of the natural history of dyslipidemia, our prevalence results are representative of how clinicians may encounter and manage dyslipidemia [37].
Implications for patient-care
Although the conventional approach of using LDL-C has been the focal point in clinical guidelines, the FLL phenotypes carry implications for morbidity and mortality and may improve dyslipidemia management. First, the majority of the lipoproteins in the phenotypes are atherogenic and associated with increased cardiovascular risk [7, 8, 38, 39]. Identifying FLL phenotypes is an opportunity to manage primary or residual cardiovascular risk in patients that appear normal under the conventional approach of only using LDL-C. This is especially important for patients with clinical or advanced subclinical atherosclerotic cardiovascular disease [40]. In isolation, LDL-C does not identify atherogenic triglyceride-enriched lipoproteins such as VLDL and remnant particles. Our data show that FLL dyslipidemias are present in 17–20% of individuals with LDL-C < 130 mg/dl, which highlights the opportunity to expand lipid management to lipoproteins beyond LDL.
Second, these phenotypes are further associated with non-cardiovascular morbidity, including life-threatening pancreatitis and pancreatic insufficiency, dermatologic manifestations (i.e. eruptive xanthomata and tendinous xanthomata), and ophthalmologic lesions (i.e. lipemia retinalis and corneal arcus) [1, 9]. These morbidities are only definitively treated by recognizing and addressing the underlying lipid disorder. The FLL phenotypes may subsequently present a framework to investigate underlying dyslipidemia in the presence of one of these clinical findings.
Third, these phenotypes are often genetic or hereditary as suggested by prior familial analyses and genetic studies [1, 9]. Although the phenotypes may be secondary to systemic conditions such as renal dysfunction and thyroid dysregulation, several of these phenotypes may be primary in nature and directly linked to deficiencies in proteins and/or enzymes involved in lipoprotein metabolism, including lipoprotein lipase, apolipoprotein CII, and apolipoprotein E among others [9, 41, 42]. Diagnosing the presence of a phenotype in an index family member may therefore help identify potential genetic causes and facilitate timely detection and treatment in other family members.
Fourth, with further lipoprotein-lowering interventions in development, dyslipidemia treatment may improve by evolving to a more personalized approach that targets specific lipoproteins. For example, in type IIa dyslipidemia, LDL is in excess and statins are the clear first line of therapy. However, in type III dyslipidemia, the excess is in TG-rich remnants and therefore statins are not clearly effective, but fibrates, omega-3 derivatives, or niacin could be indicated. More potent, novel agents in development include targets against apolipoprotein CIII and angiopoietin-like 3 and 4 to specifically affect enzymes such as lipoprotein lipase and preferentially decrease TG-rich lipoproteins [12, 43]. FLL phenotyping may consequently aid in patient selection for the selective use of current and emerging dyslipidemia therapies to reduce lifetime cumulative exposure to atherogenic lipoproteins.
Limitations and strengths
We recognize limitations of our study. The VLDL population specifically does not have information on several co-morbidities which may be associated with the FLL phenotypes. This restricted the assessment of clinical associations such as diabetes and obesity to NHANES participants.
In both study populations, chylomicrons were not directly isolated and were presumably reflected in the VLDL-C fraction. Genetic analyses and family histories for individuals were not available, limiting our assessment of primary versus secondary causes of the phenotypes. Nevertheless, given that treatment is directed at phenotypic expression of dyslipidemia, our results are clinically relevant. We further restricted our analysis to individuals 18 years of age or older to provide prevalence estimates specifically for adults. Children and adolescents may be more likely to have a primary dyslipidemia and warrant separate analysis. Our study was also restricted to individuals with fasting lipid profiles because this has been a common standard for diagnosing primary dyslipidemias and because the diagnostic algorithm that we used was derived and validated in fasting samples. The generalizability to non-fasting lipid samples, which are of increasing clinical interest, should be considered in future studies.
Strengths of our study include the complementary, cross-sectional nature of our analysis, as our results are indicative of the FLL prevalence in both the general population and those specifically referred for clinical lipid testing. To our knowledge, our study is also the first to use a validated, scalable algorithm to diagnose the FLL phenotypes in big data samples – limitations which previously prevented accurate prevalence estimation. The algorithm only requires components that are standardized by the Centers for Disease Control and Prevention (CDC) and the WHO. Specifically, the CDC Lipids Reference Laboratory provides reference values for total cholesterol and TG, whereas apoB values are provided by the Northwest Lipid Metabolism and Diabetes Research Laboratories. This standardization allows for widespread and scalable phenotyping across various clinical and laboratory settings.
In conclusion, the FLL dyslipidemia phenotypes, which are endorsed by the WHO, are likely much more prevalent than historically and clinically appreciated. This may be explained in part by the lack of large-scale studies on this topic previously as well as by the epidemics of obesity and diabetes. The high prevalence of the FLL phenotypes has important diagnostic, prognostic, and therapeutic implications. Since FLL dyslipidemia diagnosis can be automated and scaled, these results open the door for a precision medicine approach in dyslipidemia management to reduce atherosclerotic cardiovascular disease and non-cardiovascular morbidity.