Introduction
In the occurrence and development of heat stroke (HS), factors such as hyperthermia, ischemia and hypoxia are essential to the central nervous system (CNS) inflammatory response, but the main mechanism underlying CNS inflammation remains unclear [1]. Microglia are persistent sensors of microenvironmental changes in the CNS and restorers of tissue homeostasis. Along with their role as the main immune cells of the CNS, they regulate the innate immune function of other glial cells. Neuroinflammation induces the activation of molecular isomerically reactive microglia that produce deleterious (M1-type) or beneficial (M2-type) responses [2, 3]. Microglia in the healthy adult CNS are characterized by a quiescent morphology. After they acquire an activated, amoeboid morphology in response to invading pathogens and/or CNS damage they increase their production of a wide array of cytokines, reactive oxygen species and inducible nitric oxide synthase (iNOS) that induce neuroinflammation [4, 5]. At the site of CNS injuries, macrophages are derived from both microglia and hematogenous macrophages. The presence of M1/M2 phenotypic polarization has been suggested for microglia, which have been linked to functional properties including production of inflammation association molecules and phagocytic activity. Recent studies show that microglia can be polarized to a ‘classically’ activated M1 cell that is pro-inflammatory and cytotoxic, or to an ‘alternatively’ activated M2 cell that is anti-inflammatory and has reparative properties [6]. Microglia likely undergo phenotypic changes during ontogenesis, although in the healthy brain they did not exclusively express either M1 or M2 phenotypic markers at any time [7]. Studies have shown that the typical antigen markers of activated M1 microglial include CD45, CD86, CD64 and iNOS, and the microglia express high levels of the M2 markers CD206 (mannose receptor), CD163 (hemoglobin-haptoglobin scavenger receptor) and arginase 1 (Arg1) [6].
This study aimed to investigate the molecular mechanism of CNS inflammation involved in thermal injury by establishing a Beagle canine heatstroke model to analyze the expression of different types of microglia induced by thermal injury stimulation and to determine whether it could affect microglial polarization.
Material and methods
Experimental subjects and grouping
Eighteen normal grade 6-month-old Beagle dogs (male) with weights of 15 ±0.5 kg were provided by the Animal Experimental Center of Nantong University Medical College. The animal experiments in this study were approved by the ethics committee of Nantong University Medical College (Ethics council approved number: SCXK (Su): 2008-0010). Animals were kept separate from each other to ensure proper temperature for raising. All experimental procedures involving animals were in accordance with the guidelines for the use of experimental animals established by the National Health Association, and met the requirements for animal use and operation of the Animal Experimental Center of Nantong University.
All adult Beagle dogs were randomly divided into four groups. Nine animals were arranged in the normal control group (group A). The remaining animals were divided into the 1 h group of heat-radiation disease (group B), the 6 h group of heat-radiation disease according to the exposure time after thermal injury (group C), and the 24-h group of heat-shooting disease (group D), with three experimental animals in each sub-group. The animals in different heat-radiation groups were sacrificed and their hypothalamus was frozen or processed for primary microglia culture.
Main instruments and reagents
Animal temperature maintenance instrument XR200 (Xinsoft Information Technology, Shanghai) body temperature measurement setting range –30 to 60°C. Animal Blood Pressure Data Collection and Analysis System BP-2000 (Yawentai, Beijing). Fluorescence microscope (DP 71, Olympus, Japan).
Radioimmunoprecipitation test lysate (Biyuntian Bio, Shanghai); phenylmethylsulfonyl fluoride (subfamily, Suzhou); BCA protein concentration determination kit (Biyuntian Bio, Shanghai); internal reference β-actin (Ai Meijie, Wuhan); primary antibody monoclonal antibody CD45 (Aibo, Shanghai), rabbit polyclonal antibody iNOS (Aibo, Shanghai); rabbit polyclonal antibody arginase (Aibo, UK), rabbit polyclonal antibody CD206 (Amy Jie, Wuhan); phosphate buffered saline solution (Biyuntian Bio, Shanghai); secondary anti-goat anti-mouse IgG (H + L) Alexa Fluor Plus 488 (Thermo Fisher, Shanghai).
Experimental method
Beagle canine heat shock injury model
Diagnostic criteria for experimental heat-radiation disease referring to the diagnostic criteria for animal heat-radiation disease are as follows [8]: 1) core body temperature reaches 40°C with symptoms of nervous system damage such as prospecting, convulsions, and coma; 2) core temperature reaches 42.5°C or artery shows a point of decline after which the systolic blood pressure rises to a peak indicating that moderate heatstroke has occurred; 3) a severe heatstroke is considered when the core temperature reaches 43.0°C or the arterial systolic pressure drops below 90 mm Hg.
All Beagle dogs were intravenously anesthetized with 3% sodium pentobarbital (1 ml/kg) for 12 h before fasting. When the corneal reflex and pain reflex both disappeared, and the breathing and blood pressure were stable, then the anesthesia was considered satisfactory. In the control group, nine Beagle dogs were placed under an environment of 26 ±0.5°C until the end of the experiment without any other treatment. On the electric blanket of the animal temperature maintenance instrument was the place where the nine experimental group animals were arranged, and the temperature was set at 40 ±0.5°C, and the core body temperature (rectal temperature) was monitored every 5 min. When the core body temperature reached 40°C, the observation continued, while the average arterial pressure measurements were initiated. Falling of the arterial pressure as a time node is considered successful for thermal imaging modeling. After successful modeling, all experimental animals were transferred to an environment of 26 ±0.5°C and 60 ±0.5% humidity, and routinely bred, with ECG and hemodynamic monitoring performed simultaneously.
As shown in Figures 1 A and 1 B, the Beagle dogs were placed on the operating table for 1 h, 6 h, and 24 h with the head fixed in a lateral position, the ear edge fixed to the caudal side, and anesthesia was administered. The craniotomy was performed through a sacral approach. After finishing craniotomy, the subcutaneous tissue was turned to the lateral bone flap, and the bone window was as close as possible to the skull base. After washing off excess blood with physiological saline, brain tissue was placed in a tube and stored at –80°C for later use. Next, 100 mg of brain tissue was used to prepare 1 ml of cell lysate, homogenized, centrifuged, and the supernatant aspirated. After adding a portion of the protein buffer and boiling for 15 min, western blot was performed. The supernatant of the remaining cells was placed at –80°C for future use.
Figure 1
Beagle canine heat shock injury model. A – The Beagle dog is placed in the lateral position, the head is rotated to the opposite side, and the ear edge is pulled toward the midline and the caudal side. B – Craniotomy was performed through a sacral approach. After the craniotomy was fully performed, the subcutaneous tissue was turned to the lateral bone flap, and the bone window was as close as possible to the skull base. C – Primary microglia (100 ×, magnification)
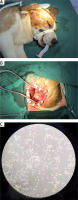
Western blot analysis of microglia-specific protein marker expression
The brain tissues of the normal group and different time points after thermal injury were examined by western blot, and the expression of CD45, iNOS, arginase, and CD206 in the 4 groups of microglia was observed. Protein was quantified by the BCA assay, separated by polyacrylamide gel electrophoresis, and then transferred to a polyvinylidene fluoride membrane, followed by blocking with 5% Tris-Cl (pH 6.8) containing 5% skim milk powder at room temperature for 1 h. After TBST rinse, antibodies against CD45, iNOS, arginase, CD206 and β-actin antibody were incubated overnight at 4°C, rinsed 5 times with TBST for 5 min, and then incubated with HRP-labeled secondary antibody for 2 h at room temperature. Following rinses 3 times with TBST for 15 min, chemiluminescent substrate was added followed by dark room exposure and development. Strip gray values were determined using Image J analysis.
Immunofluorescence analysis
The hypothalamic tissues from three groups were trypsinized to extract the primary microglia and cell morphology was observed under an electron microscope (Figure 1 C), then cells were transferred to a Petri dish and cultured with an appropriate amount of medium. Immunofluorescence analysis was used to detect the expression of CD45 cells and arginase immunoreactive cells after heat exposure stimulation. The stabilized nerve cells were cultured with medium discarded. Then, cells were rinsed with PBS, fixed in 4% paraformaldehyde for 40 min at room temperature, rinsed 3 times with PBS for 10 min, and then incubated with fluorescent blocking solution for 2 h at room temperature. After completion, CD45 antibody (microglia M1 type), arginase antibody (microglia M2 type) was incubated overnight at 4°C, rinsed 3 times with PBS for 5 min, and stained with Dylight 594 (1 : 400) and Dylight 488 (1 : 400) fluorescent labels. The secondary antibody was incubated at room temperature for 2 h in the dark, and rinsed another 3 times with PBS for 5 min, DAPI counterstain was added for 30 min in the dark, then it was rinsed again 3 times with PBS for 15 min, and then sealed with fluorescent sealing liquid. Cells were observed and photographed using a confocal microscope. Fusion image overlay showing double-marked cells was obtained.
Results
CD45 and iNOS protein expression in Beagle dogs
The M1 type specific marker proteins CD45 and iNOS were detected by western blot in the hypothalamus of experimental animals from the four groups. A group of CD45 and iNOS proteins was detected in group A. The levels of the two protein markers in group B were significantly higher than those in group A (p < 0.05), and the protein marker levels in group C were still higher than those in group A (p < 0.05). There was no significant difference among the animals in group A (p > 0.05). It was suggested that the expression of CD45 and iNOS was increased significantly at 1 h after thermal injury, gradually decreased after 6 h and returned to the normal control level after 24 h (Figures 2 A and 2 B).
Figure 2
M1/M2 protein expression of microglia after heat injury in each group. A – is the normal control group; B – is the 1 h group after thermal injury; C – is 6 h group; D – is 24 h group. CD45 – leukocyte differentiation antigen 45, iNOS – inducible nitric oxide synthase, CD206 – leukocyte differentiation antigen 206. A – The expression of M1/M2 specific protein in microglia by western blot after thermal injury in Beagle dogs. B – The results of corresponding western blot analysis
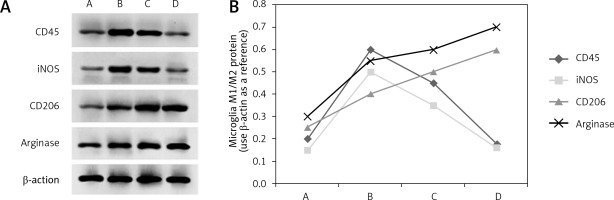
Arginase and CD206 protein expression in Beagle dogs
M2 type specific markers arginase and CD206 were detected in the brain tissue of experimental animals from four groups. A group of arginase and CD206 proteins was detected in group A. The levels of the two protein markers in group B were much higher than those in group A (p < 0.05), and the protein marker levels in group C were even higher than those in group A (p < 0.05). Further analysis was then performed. Levels of both protein markers were significantly higher in group D than in group A (p < 0.001). It was thus suggested that the expression of arginase and CD206 proteins began to increase at 1 h after thermal injury and reached a peak after 24 h (Figures 2 A and 2 B).
Immunofluorescence co-localization of M1/M2 marker expression
As shown by immunofluorescence, CD45 showed a red fluorescent label and arginase showed a green fluorescent label. CD45 and arginase showed low expression in the normal control group. We observed the immunofluorescence of each subgroup after heat shock: thermal injury for 1 h (group B), 6 h (group C), and 24 h (group D). A small amount of red fluorescence was observed in group B and group D, and a significant amount of red fluorescence was observed in group C, which was different from group B and group D (p < 0.001), suggesting that the expression of CD45 was increased after 6 h of thermal injury. In addition, the green fluorescence of group C was higher than that of group B (p < 0.001), while that of group D was the most obvious (p < 0.001). It suggested that the expression of arginase increased 6 h after heat injury, and the most obvious increase was 24 h after heat injury (Figure 3).
Discussion
Heat stroke is a serious clinical symptom caused by elevated core temperature, from which patients will suffer from varying degrees of CNS damage. The vulnerable areas in the CNS are mainly in the hypothalamus, cerebellum, and hippocampus [9]. Related studies have indicated that hyperthermia and neuroinflammatory reactions are the main causes of central brain injury in HS patients. Although early active treatment can be achieved to save the lives of most HS patients, one-third of these patients will display permanent nerve damage [10]. At the early stage of HS, cerebral edema will occur, which is mostly reversible compared with irreversible cerebral edema caused by trauma and cerebral hemorrhage and embolism [11]. Once stimulated by various pathological stimuli, microglia in the CNS will be fleetly activated. Afterwards, microglia will mainly manifest two phenotypes: M1 type microglia that mainly secrete pro-inflammatory factors such as IL-1β, IL-6, TNF-α, and NO, exerting neurotoxic effects including promoting inflammation and inhibiting phagocytosis; and M2 type microglia that mainly secrete anti-inflammatory factors, transforming growth factor β (TGF-β), arginase, and neurotrophic factors with neuroprotective effects including regeneration. The relationship between M1/M2 polarization trends and early brain injury in heat-induced disease is the key to CNS injury in thermal radiation.
Previously, mice were mostly used as the thermal injection model [12–14]. However, in the present study, Beagle dogs were chosen to be the model subjects, considering that large animals such as dogs can be installed with ECG monitoring and hemodynamic monitoring to obtain real-time data. Moreover, more pathophysiological data can be acquired after changing the environmental setting parameters and dynamically observing the animal activity parameters. The expression of M-type polarizing markers CD45 and iNOS in 1–6 h microglia reached a peak at 6 h, and gradually decreased during the recovery time, then returned to normal at 24 h. The expression levels of M2-type polarized markers arginase and CD206 began to increase gradually from 1 to 6 h after HS, and reached the highest level after 24 h. Furthermore, the expression of M1 and M2 activated phenotype molecules in microglia was observed by immunofluorescence-labeled laser confocal microscopy. The M1 activation marker was marked at 6 h after heat shock, but it was significantly weakened after 24 h. The M2 activation marker became active 6 h after HS and was clearly marked at 24 h. The results indicated that M1 type microglia were mainly activated in the early 1–6 h of CNS injury and M2 type microglia were mainly activated after 6–24 h, M2 being dominant at 24 h. Biedenkapp et al. [15] established a mouse thermal radiation model to observe the changes in gene expression of various cytokines and chemical factors in a recovery period within 24 h, and found that the expression of inflammation-related factor genes began to increase significantly within 2 h after heat-induced disease and returned to the control level at 24 h, which was similar to our experimental results. In this experiment, we chose 1 h, 6 h, and 24 h as the phase observation points. In the future, we will continue to expand the animal sample size and observe the dynamic changes in the central nervous microglia in an hourly manner.
Studies have shown that pre-heavy and light, with gradual recovery are the main characteristics of the CNS inflammatory response and HE histopathological changes within 24 h after thermal injury [16]. This trend is in line with the M1/M2 polarization trend of microglia. At the early stage of heat radiation, small glial cells gradually become polarized to M2 type, and their anti-inflammatory and prosthetic effects result in heat-induced disease. The CNS inflammatory response and histopathological damage are gradually alleviated and restored. Therefore, microglia may be one of the important targets of thermal stimulation-mediated CNS injury, and regulating their polarization by limiting M1 or promoting M2 activation may become a potential strategy for the treatment of brain damage caused by heat-induced diseases.
In CNS diseases such as Parkinson’s disease, Alzheimer’s disease, atrophic lateral sclerosis, multiple sclerosis, and stroke, microglia can rapidly develop an early inflammatory reaction, followed by an astrocyte reaction [17–20]. Molecular dialogue among glial cells provides an important and novel direction for the CNS to participate in health and disease [21, 22]. In a new era of glial cell biology, future research is needed to expand our understanding of the importance of crosstalk between resident and infiltrating immune cells in neurons, astrocytes, and the CNS [23]. Therefore, whether astrocytes play a role in the CNS inflammatory response of heat-induced disease is a point worthy of further study.