Introduction
Sepsis is a systemic disease characterized by an uncontrolled response to infection leading to life-threatening multiple-organ dysfunction [1]. The cardiac system is frequently affected during sepsis, with cardiovascular dysfunction observed in more than 40% of patients and a mortality rate as high as 70% [2]. This may involve key inflammatory factors in the cardiac and peripheral system as well as apoptosis of cardiomyocyte apoptosis [3]. Even though fluid resuscitation, antibiotic therapy, and vascular compression are actively used in the management of patients with sepsis, their prognosis remains unsatisfactory [4]. As a disease with rapid onset and progression, cardiac dysfunction is the most serious complication in the development of sepsis, which directly affects the prognosis of patients, and there is no specific drug. Therefore, early diagnosis of cardiac dysfunction in patients with sepsis can facilitate prompt treatment, mitigate disease progression, and prolong survival [5].
Long non-coding RNAs (lncRNAs) are transcripts containing more than 200 nt with limited or no protein-coding potential. They can regulate gene expression and participate in disease progression and drug resistance through transcriptional or post-transcriptional regulation [6–9]. Additionally, accumulating evidence has focused on the potential of lncRNA as a biomarker because it is present in body fluids (blood, urine) with non-invasive and less time-consuming detection [10]. For example, LncRNA CASC15 [11], GSEC [12], and MEG3 [13] are biomarkers of sepsis and are associated with inflammation. LncRNA small nucleolar RNA host gene 8 (SNHG8), located on 4q26, is a novel small nucleolar guide RNA. Previous studies have emphasized its overexpression in a variety of cancers [14]. SNHG8 was identified as a key regulator of myocardial infarction [15] and participates in the release of CK-MB, cTnI, and the expression of inflammatory factors in hypoxia-induced myocardial injury [16]. SNHG8 serves as a diagnostic marker for atherosclerosis and facilitates the proliferation of vascular smooth muscle cells through sponging miR-224-3p [17]. In terms of inflammation, elevated SNHG8 decreased the production of inflammatory factors in periodontal cells [18] and attenuated microglia inflammation and blood-brain barrier damage in ischemic stroke [19]. Interestingly, Guo et al. identified lncRNAs associated with sepsis by lncRNA profiling from sepsis and healthy controls, including SNHG8 [20]. The current body of research on SNHG8 in sepsis is insufficient.
To fill this gap, we explored for the first time the clinical significance of serum SNHG8 levels in sepsis and investigate its potential function in sepsis cardiac dysfunction and inflammation by mediating SNHG8 expression. The present study showed for the first time that SNHG8 not only has the potential to serve as a diagnostic biomarker for sepsis but also predicts cardiac insufficiency in sepsis. Finally, the molecular mechanism by which SNHG8 affects sepsis was explored by LPS induction. This may guide the effective diagnosis and management of sepsis in the future.
Material and methods
Subjects in this research
One hundred and twenty-six consecutive patients with sepsis were treated in the ICU between January 2018 and June 2021 in Binzhou Medical University Hospital and 81 healthy individuals as the controls who underwent a health examination during the same period were consecutively recruited. Patients with sepsis were based on the following recruitment criteria: meeting the international diagnostic criteria for sepsis published by the SCCM/ESICM in 2016; age ≥ 18 years; less than 48 h from diagnosis to ICU admission. The exclusion criteria were as follows: pregnant or lactating women; patients with immunodeficiency or recent (within 3 months) immunosuppression; patients with hepatic (serum bilirubin, aspartate transaminase, alanine transaminase, and alkaline phosphatase > 1.5 times the upper limit of normal) or renal [serum creatinine ≥ 1.5 mg/dl (men) or ≥ 1.4 mg/dl (women)] insufficiency; malignancies; history of recent myocardial infarction; post-operative cardiac patients. The patient’s blood was collected within 24 h of admission, while the controls were collected on the day of enrollment. Laboratory parameters such as serum creatinine (Scr), albumin, white blood cells (WBC), and procalcitonin (PCT) were recorded along with age, gender, and body mass index (BMI). The patient’s APACHE II and SOFA scores were also classified in the first 24 h after ICU admission. What is more, left ventricular ejection fraction (LVEF) levels were measured, and LVEF < 50% was defined as a diagnostic criterion for cardiac dysfunction. Then, patients with sepsis were divided into cardiac dysfunction and non-cardiac dysfunction groups.
Cell culture and lipopolysaccharide (LPS) induction
Human ventricular cardiomyocytes of the AC16 cell line were purchased from the BeNa Culture Collection (Henan, China) and cultured in DMEM supplemented with 10% FBS and 1% penicillin/streptomycin at 37°C under 5% CO2. To construct a sepsis-associated cardiac dysfunction cell model, AC16 cells were exposed to a concentration gradient of 0, 1, 5, and 10 μg/ml LPS for 24 h [21].
Cell transfection
The SNHG8 overexpression pcDNA3.1 plasmid (Ov-SNHG8) and pcDNA3.1 empty plasmid (Ov-Ctrl) were synthesized by GenePharma (Shanghai, China). miR-34b-5p mimic, miR-34b-5p inhibitor, mimic NC, and inhibitor NC were obtained from RiboBio (Guangzhou, China). Logarithmic growth stage AC16 was inoculated in 6-well plates and transfected following LPS induction with the Lipofectamine 3000 transfection reagent.
Real-time quantitative reverse transcription PCR (RT-qPCR)
Total RNA was purified from serum and cell lines with Trizol reagent extracted and dissolved in 30 μl of diethylpyrocarbonate (DEPC)-treated water. The concentration and purity of RNA were confirmed by the NanoDrop 2000 Spectrophotometer. Subsequently, 500 ng of RNA was reverse transcribed to cDNA using the RNA PrimeScript RT reagent Kit or mir-X miRNA First Strand Synthesis Kit. The primers were derived using Primer Premier 5.0 software and synthesized by GENEWIZ (Suzhou, China). The primer sequences used in the qPCR are shown in Table I. Next, cDNA, primers, and TB Green Fast qPCR Mix kit or mir-X miRNA qRT-PCR SYBR Kit were mixed, and the PCR amplification reaction performed on an ABI 7500 qPCR instrument. U6 and GAPDH were employed as the endogenous references for miR-34b-5p and SNHG8, respectively. The 2−ΔΔCt method was used to calculate the relative expression levels. False-positive and false-negative results were minimized by performing three biological replicates per sample and by setting up internal reference genes.
Enzyme-linked immunosorbent assay (ELISA)
A commercial ELISA kit was employed to detect inflammatory factor levels in the patient’s serum and cell supernatants. 96-well plates were coated with IL-6, TNF-α, and IL-1β antibodies for 4 h at 37°C. After being washed with PBS and removed, 200 μl of diluted 5% skimmed milk was added to seal the plates. The 96-well plates were washed after 2 h and diluted standard and experimental samples were added. Incubation was performed at 37°C for 1.5 h and diluted antibody was added at room temperature for 2 h. Affinity-horseradish peroxidase was added and incubated at room temperature for 2 h. After incubation with tetramethyl benzidine (TMB) at room temperature for 15–35 min, the optical density (OD) at 405 nm was measured and the protein concentration was determined according to the standard curve.
Cell viability assay
LPS-induced and transfected AC16 cells were inoculated into 96-well plates at 5 × 103 cells/well. 10 μl of Cell Counting Kit (CCK)-8 reagent was mixed with 90 μl of DMEM. The medium in the cells was replaced with this mixture at every 24 h interval. The OD value at 450 nm was measured after 1 h incubation in the incubator. The cell supernatants of 96-well plates were observed to show different shades of orange-yellow color. The OD values at 450 nm were measured, and the cell viability changes were counted after 4 consecutive days.
Apoptosis analysis
Flow cytometry and apoptosis detection kits were employed to examine cell apoptosis. The LPS-induced and transfected cells were digested in EDTA-free trypsin, washed with pre-cooled PBS at 4°C, and 200 μl of binding buffer was added to the cells. 5 μl of Annexin V-FITC and 5 μl of PI were mixed with the cell suspension and passed through a 200-mesh sieve, and the number of apoptotic cells was detected by flow cytometer.
Nuclear-cytoplasmic fractionation assay
Distribution of SNHG8 in the nuclear and cytoplasmic fractions was measured by the PARIS kit (AM1921, Invitrogen, USA). Briefly, AC16 was lysed in a pre-cooled cell fraction buffer for 10 min. The supernatant was collected as the cytoplasmic fraction after centrifugation at 500 g for 3 min at 4°C. Then, the precipitated fraction was washed with cell fraction buffer, and a mixture of equal volumes of ethanol and lysis/binding buffer was added. RT-qPCR was performed to quantify the nuclear and cytoplasmic fractions of SNHG8, using U6 and GAPDH as internal references in the nucleus and cytoplasm, respectively.
Dual-luciferase reporter gene assay
Prediction of target miRNAs for SNHG8 in ENCORI (http://starbase.sysu.edu.cn) and identification of miR-34b-5p binding sites were performed. SNHG8 wild-type (WT) and SNHG8-mutant (Mut) plasmids were subcloned into the luciferase reporter plasmid pGL3 basic luciferase vector (Promega, USA). AC16 cells were inoculated into 24-well plates, and the above plasmids were co-transfected into cells with miR-34b-5p mimic, miR-34b-5p inhibitor, mimic NC, and inhibitor NC with the help of transfection reagent Lipofectamine 3000. After 48 h, the relative luciferase activity was detected by a dual-luciferase reporter assay kit and normalized to Renilla luciferase activity.
RNA immunoprecipitation (RIP) assay
The endogenous association between cell viability, SNHG8 and miR-34b-5p was identified by RIP with Magna RNA binding protein immunoprecipitation kits. Cells were centrifuged at 3000 g for 10 min and resuspended by adding 100 μl of RIPA lysis buffer. 50 μl of magnetic beads containing Ago2 antibody or negative control IgG were mixed with the lysed cell resuspension and incubated overnight at 4°C on a shaker. RT-qPCR was used to examine the expression of SNHG8 and miR-34b-5p.
Statistical analysis
The data were statistically analyzed using SPSS 23.0 and GraphPad Prism 6. The Shapiro-Wilk test was used to assess the normality of the variable distribution. Continuous variables were compared using unpaired Student’s t-test and one-way analysis of variance (ANOVA) followed by Tukey’s post-hoc test. Normally distributed data were expressed as mean ± SD. Non-normally distributed data were expressed as median (first quartile, third quartile), and the Mann-Whitney U test was used to analyze statistical differences. The count data are presented in the form of a number (%) and compared with the χ2 test. Receiver operating characteristic curves (ROC) diagnosis was performed to assess the diagnostic value. Correlation analysis was conducted by Pearson correlation analysis. In vitro experiments were averaged from three independent experiments. Probability levels of ≤ 0.05 were presented to represent statistical significance.
Results
Characteristics of sepsis patients
Detailed information on baseline characteristics is illustrated in Table II. Patients with sepsis showed no significant differences from the controls in terms of age, gender, BMI, and current smoking (p > 0.05). However, the Scr, WBC, PCT, C-reactive protein (CRP), and inflammatory factors (IL-6, IL-8, and TNF-α) were typically higher in the sepsis patients than in controls (p < 0.05). The results suggest that sepsis patients are representative.
Table II
Clinical characteristics of subjects
[i] BMI – body mass index, Scr – serum creatinine, WBC – white blood cell count, CRP – C-reactive protein, PCT – procalcitonin, IL-6 – interleukin 6, TNF-α – tumor necrosis factor α. Data are presented as mean ± SD for normally distributed continuous variables; median and interquartile range (first quartile, third quartile) for non-normally distributed and n% for categorical variables.
Reduced SNHG8 levels represent a potential diagnostic biomarker for sepsis
The levels of SNHG8 in the serum of the subjects were explored. RT-qPCR confirmed that SNHG8 was typically lower in sepsis patients than the controls (p < 0.01, Figure 1 A). Additionally, the levels of PCT, a biomarker of sepsis caused by a bacterial infection in sepsis, were negatively correlated with SNHG8 (r = –0.716, p < 0.001, Figure 1 B). ROC curve analysis revealed that SNHG8 had a sensitivity of 87.65% and specificity of 78.57% for identifying patients with sepsis (AUC = 0.878, 95% CI = 0.827–0.929, Figure 1 C). The findings confirmed that reduced SNHG8 might be a valuable diagnostic biomarker in patients with sepsis.
Figure 1
Serum SNHT8 level and its diagnostic value in patients with sepsis. A – RT-qPCR was performed to examine serum SNHG8 levels in patients with sepsis. B – Pearson correlation analysis was employed to explore the correlation between serum SNHG8 levels and PCT in patients with sepsis. C – The diagnostic value of SNHG8 in sepsis was analyzed by the ROC and its AUC. ***P < 0.001, vs. controls.
ROC – receiver operating characteristic, AUC – area under the curve.
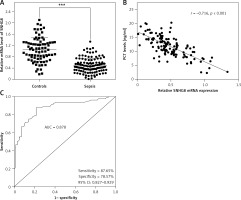
Serum SNHG8 levels were negatively correlated with CRP and inflammatory factors
As an inflammatory disease, we also analyzed the correlation of CRP and inflammatory factor levels with serum SNHG8 in patients with sepsis. As illustrated in Figure 2 A, CRP was negatively correlated with serum SNHG8 levels (r = –0.651, p < 0.001). Meanwhile, the inflammatory factors IL-6 (r = –0.644), TNF-α (r = –0.652), and IL-8 (r = –0.661) were negatively correlated with SNHG8 levels (p < 0.001, Figures 2 B–D). These findings indicate that SNHG8 may be implicated in the inflammation caused by sepsis.
SNHG8 participates in the development of cardiac dysfunction in sepsis
To further analyze the potential role of SNHG8 in the development of cardiac dysfunction, all sepsis patients were grouped according to their cardiac function. Among them, 74 patients presented with cardiac dysfunction, and 52 patients had normal cardiac function. The clinical baseline characteristics of the two groups were compared. There were no significant differences in age, gender, BMI, smoking, primary infection site, or primary organism (p > 0.05), but levels of MAP, WBC, PCT, CRP, and inflammatory factors (IL-6, IL-8, and TNF-α) were significantly higher in the cardiac dysfunction group (p < 0.05, Table III). Patients with cardiac dysfunction experienced more coronary heart disease (CHD). RT-qPCR analysis confirmed that serum SNHG8 levels were significantly lower in sepsis patients who had cardiac dysfunction than those without cardiac dysfunction (p < 0.05, Figure 3 A).
Table III
Comparison of clinical data in patients with cardiac dysfunction and non-cardiac dysfunction
[i] BMI – body mass index, MAP – mean arterial pressure, HR – heart rate, Scr – serum creatinine, WBC – white blood cell count, CRP – C-reactive protein, cTnT – cardiac troponin T, IL-6 – interleukin 6, TNF-α – tumor necrosis factor α, CHB – coronary heart disease. Data are presented as mean ± SD for normally distributed continuous variables; median and interquartile range (first quartile, third quartile) for non-normally distributed and n% for categorical variables.
Figure 3
Expression levels of SNHG8 levels in sepsis with cardiac dysfunction (A) and ROC curve predicting sepsis cardiac dysfunction based on its level (B). *** P < 0.001, vs. controls
ROC – receiver operating characteristic, AUC – area under the curve.
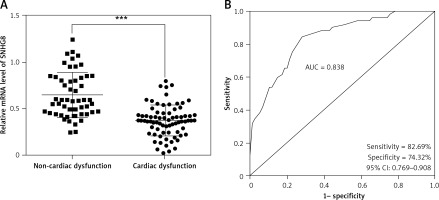
Potential factors influencing cardiac dysfunction in patients with sepsis were identified by logistic regression analysis. The results confirmed that SNHG8 (HR = 5.466, 95% CI = 2.230–13.397, p < 0.001, Table IV) was an independent factor influencing cardiac dysfunction. Moreover, the ROC curve showed that the sensitivity and specificity of SNHG8 in predicting cardiac dysfunction in sepsis were 82.69% and 74.32%, respectively (Figure 3 B).
Table IV
Logistic regression analysis of factors associated with occurrence of cardiac dysfunction in patients with sepsis
Elevated SNHG8 alleviated LPS-induced cardiomyocyte apoptosis and increased inflammation
To further investigate the potential effect of SNHG8 on cardiac dysfunction and inflammation in sepsis, an in vitro model of sepsis induced by LPS was constructed. As illustrated in Figures 4 A, B, SNHG8 decreased in a dose- and time-dependent manner with the increase of LPS concentration and induction time (p < 0.05). Cells were induced with LPS (10 μg/ml) for 24 h for subsequent studies. Overexpression of SNHG8 significantly restored SNHG8 levels after LPS induction (p < 0.05, Figure 4 C). CCK-8 confirmed that LPS reduced cardiomyocyte proliferation, but this reduction was suppressed by SNHG8 overexpression (p < 0.05, Figure 4 D). Additionally, LPS promoted cell apoptosis, which was also reversed by SNHG8 overexpression (p < 0.05, Figure 4 E). Finally, SNHG8 significantly inhibited the release of pro-inflammatory factors induced by LPS in cardiomyocytes (p < 0.05, Figure 4 F).
Figure 4
SNHG8 regulates proliferation, apoptosis, and the inflammatory response. RT-qPCR was employed to examine the SNHG8 levels in cardiomyocytes induced by different LPS concentrations for 24 h (A) or 10 μg/ml LPS at different times (B). C – RT-qPCR was performed to analyze SNHG8 levels in LPS-induced cardiomyocytes induced by LPS after transfection with overexpressed SNHG8 plasmid (ov-SNHG8). CCK-8, flow cytometry, and ELISA were used to examine the role of SNHG8 in the proliferation (D), apoptosis (E), and secretion of inflammatory factors (F) in cardiomyocytes induced by LPS. *P < 0.05, **p < 0.01, ***p < 0.001 vs. control; #P < 0.05, ##p < 0.01, ###p < 0.001 vs. LPS + ov-Ctrl
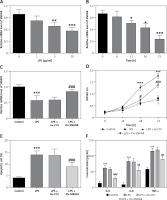
SNHG8 sponges miR-34b-5p in sepsis
The underlying molecular mechanism of SNHG8 in sepsis was further explored. Subcellular isolation expression confirmed that SNHG8 was more enriched in the cytoplasm than in the nucleus (p < 0.05, Figure 5 A), which verified the possibility of a competing endogenous RNA (ceRNA) mechanism (p < 0.05, Figure 5 B). RIP assay confirmed that SNHG8 and miR-34b-5p expression was enriched in the anti-Ago2 group. The potential binding sequence of SNHG8 and miR-34b-5p is illustrated in Figure 5 C. More importantly, miR-34b-5p mimic decreased the luciferase activity of SNHG8-WT, while miR-34b-5p inhibitor increased the luciferase activity (p < 0.05), but they did not significantly change SNHG8-MUT luciferase activity, verifying that SNHG8 targets miR-34b-5p (p > 0.05, Figure 5 D). Finally, serum miR-34b-5p was significantly elevated in patients with sepsis (p < 0.05, Figure 5 E) and negatively correlated with SNHG8 levels (p < 0.05, Figure 5 F).
Figure 5
miR-34b-5p was the target miRNA of SNHG8. A – Subcellular isolation assay was used for localization of SNHG8. B – RIP assay examined relative RNA expression. C – Binding sites between SNHG8 and miR-34b-5p. D – Luciferase reporter assay verified the putative binding sites between SNHG8 and miR-34b-5p. E – RT-qPCR employed the serum levels of miR-34b-5p in patients with sepsis. F – Pearson correlation was performed to examine the correlation between miR-34b-5p and SNHG8 in patients with sepsis. ***P < 0.001 vs anti-IgG or mimic NC or controls
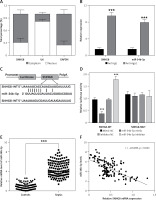
Discussion
This study investigated the diagnostic significance of SNHG8 in sepsis and the potential relationship between inflammation and cardiac dysfunction. SNHG8 was confirmed to be reduced in sepsis and further decreased in sepsis-related cardiac dysfunction. SNHG8 may serve as a potential diagnostic marker for sepsis and also hold predictive value for cardiac dysfunction. SNHG8 can regulate inflammatory factor secretion and cardiomyocyte apoptosis by sponging miR-34b-5p.
The diagnostic and prognostic value of several biomarkers, such as CRP as an inflammatory marker and PCT as a marker of bacteremia, have been widely utilized as acute phase reactants in critically ill patients [22], yet the results are suboptimal [23]. Recently, the qSOFA score was introduced as a bedside standard based on three clinical elements [24]. However, some studies have reported that qSOFA exhibits high specificity but low sensitivity and is inadequate for the early detection of patients in the disease course [25]. The search for optimal biomarkers is currently underway; however, progress in this area has been notably sluggish [26, 27]. Therefore, proactive search and exploration for ideal biomarkers of sepsis are essential. An early study reported upregulation of SNHG8 in patients with atherosclerosis and with certain diagnostic values [17]. Additionally, SNHG8 was significantly reduced in hippocampal tissue in chronic cerebral ischemia [28], and significantly elevated in hypoxic-ischemic-reoxygenation-induced myocardial injury [29] and acute gouty arthritis [30]. In contrast, in the present study, we found that SNHG8 was markedly suppressed in sepsis patients, which is consistent with the results of Guo et al. screening for differentially expressed LncRNAs in sepsis [20]. We discovered that SNHG8 levels were negatively associated with the current common diagnostic biomarker PCT. ROC confirmed that SNHG8 identifies sepsis patients from healthy individuals, demonstrating a high diagnostic value.
Studies on SNHG8 function have focused on the inflammatory response. SNHG8 inhibits the inflammatory response, negatively regulates the NF-κB signaling pathway [18], and participates in the microglia inflammatory response in cerebral ischemic stroke through sponging miR-425-5p [19]. In myocardial injury, elevated SNHG8 ameliorates hypoxia-induced elevated levels of pro-inflammatory factors TNF-α, IL-1β, and IL-6 expression [16]. Sepsis is an inflammatory disease [31], and the present study confirmed that elevated serum levels of SNHG8 were negatively correlated with CRP and pro-inflammatory factors (IL-6, IL-8, and TNF-α). It is suggested that SNHG8 may be involved in the inflammatory response in sepsis.
Myocardial tissue is frequently targeted during sepsis, and injury to this tissue marks the initial stage of syndromes characterized by multi-organ dysfunction [32]. Previous studies have confirmed that the incidence of cardiac damage and cardiac insufficiency in patients with sepsis can be as high as 40–50% and that the presence of cardiac dysfunction in patients with sepsis increases mortality from 20% to 70% [33]. To address the association between SNHG8 and cardiac dysfunction in sepsis patients, we classified sepsis patients into a normal cardiac function group and a dysfunctional cardiac function group. Seventy-four patients had cardiac dysfunction, and SNHG8 was typically downregulated. What is more, to analyze the potential factors influencing affecting cardiac dysfunction, logistic regression analysis was performed and confirmed that SNHG8 was an independent predictor of the development of cardiac dysfunction in patients with sepsis and was significant in the identification of cardiac dysfunction in patients with sepsis. After confirming the clinical diagnostic value of SNHG8 in sepsis and cardiac dysfunction, we suspected that SNHG8 might regulate the inflammatory and cardiac dysfunction processes of sepsis.
LPS is a component of the cell wall of gram-negative bacteria and is an essential initiating factor in sepsis [34]. In the present study, an in vitro sepsis model was constructed by inducing cardiomyocyte AC16 cells with LPS, and SNHG8 levels were found to decrease with increasing doses of LPS concentration and induction time. The inhibitory effect of LPS on cardiomyocyte proliferation was significantly attenuated by the upregulation of SNHG8. Excessive activation of cardiomyocyte apoptosis is an important characteristic of sepsis-induced cardiac dysfunction, while the overproduction of inflammatory responses serves as a molecular mechanism for inhibiting cardiac function [35]. Here, we confirmed that LPS increased apoptosis of cardiomyocytes and promoted the secretion of inflammatory factors, but elevated SNHG8 reversed this increase. Our study suggests that SNHG8 is involved in sepsis progression by regulating inflammation and cardiomyocyte proliferation and apoptosis.
In general, the function of lncRNAs is mainly determined by their subcellular localization. LncRNAs localized in the cytoplasm act through the regulatory mechanisms of ceRNAs, and sponge specific miRNAs to restore the expression and regulation of target genes [36]. To further explore the mechanism of SNHG8 in sepsis, we first performed subcellular localization of SNHG8 and confirmed its presence in the cytoplasm. What is more, miR-34b-5p was also found to be the target miRNA of SNHG8. Some reports have suggested that miR-34b-5p is implicated in sepsis-induced organ injury, and overexpression of miR-34b-5p worsens the inflammatory response in acute kidney injury in sepsis [37] and promotes sepsis-induced acute lung injury [38]. Inhibition of miR-34b-5p can weaken the inflammation and apoptosis of cardiomyocytes stimulated by LPS and play a protective role in cardiomyocytes [39]. Consistent with the above studies, the current study found that miR-34b-5p was elevated in sepsis. We also found that miR-34b-5p levels were negatively associated with SNHG8 in sepsis patients. Finally, we tried to explore the downstream genes of miR-34b-5p through TargetScan, miRDB, miRWalk, ECORI, DIANA, and miRTarBase database prediction and Venn diagram analysis. Ten overlapping target genes were identified, including SLC29A1, CAV1, MYC, XRCC6, MAP3K7, MET, CREB1, MTDH, HOXC8 and CAMSAP2 (Supplementary Figure S1). Among them, studies have found that MYC is significantly downregulated in sepsis and ARDS and is related to immune infiltration. Also, targeted binding of miR-34b-5p to MYC has been demonstrated in clear cell renal cell carcinoma [40], colitis-associated cancer [41], and diffuse large B-cell lymphoma [42]. However, whether SNHG8/miR-34b-5p is involved in sepsis progression through MYC still needs to be further explored.
This study does have several limitations that need to be considered when interpreting the findings. As a preliminary study, the sample size limits the generalizability of our data. The definition of cardiac dysfunction as LVEF < 50% may be inadequate, so a more stringent definition will be used for analysis in subsequent in-depth studies. Furthermore, although it has been reported that miR-34b-5p exerts a cardioprotective effect in an in vitro cellular model of LPS-induced sepsis, the current preliminary study lacks functional validation of miR-34b-5p in cardiac dysfunction in experiments in vivo and in vitro, which will also be addressed in further studies. Furthermore, the downstream target gene and related signaling pathways activated or inhibited by the SNHG8/miR-34b-5p axis action were not addressed in this preliminary study, but will be investigated in our subsequent studies. Finally, although we found that SNHG8 has some diagnostic value to identify sepsis and is negatively correlated with the common diagnostic index PCT, whether SNHG8 can be combined with it to assist in sepsis diagnosis needs to be further tested in clinical practice.
In conclusion, taking the evidence together, it was found for the first time that the SNHG8 level was reduced in sepsis, it is a potential diagnostic biomarker for sepsis and it can predict the onset of cardiac dysfunction. SNHG8 may participate in the progression of sepsis through the regulation of cardiomyocyte inflammation and apoptosis by adsorption of miR-34b-5p. Our study opens up new perspectives on the diagnosis and treatment of sepsis.