Introduction
Neonatal jaundice is a common condition worldwide, which may be physiological or pathological. Physiological jaundice results from hyperbilirubinemia, which presents as a high bilirubin concentration in the serum. Total serum bilirubin (TSB) is currently used as a diagnostic index for jaundice [1]. Most bilirubin comes from red blood cells (RBCs), which undergo hemolysis and release their hemoglobin content, with unconjugated bilirubin being the end-product of hemoglobin metabolism. After binding to serum albumin, unconjugated bilirubin is transported to the liver, where it will detach from the albumin and conjugate with glucuronic acid [2]. Conjugated bilirubin is water soluble and excreted into the small intestine with bile acids and then is further catabolized by colonic flora to urobilinogen, which is excreted from the body [3].
However, newborns have an increased bilirubin production because of the shorter lifespan of their RBCs and higher concentration of hemoglobin. On the other hand, newborns have a poorer capacity for the clearance of bilirubin. At birth, infants also have significantly lower levels of plasma albumin compared to adults [4]. The non-albumin-bound unconjugated bilirubin will pass through the blood-brain barrier and result in neuronal injury [5]. In the small intestine, the flora converts bilirubin monoglucuronides to urobilinogen, which is excreted from the body. In addition, the metabolites produced by intestinal microbiota contribute to the motility of the gastrointestinal tract (GIT). Nevertheless, newborns lack intestinal flora, and thus have a decreased intestinal motility [6]. Thus, a proportion of the bilirubin is deconjugated by β-glucuronidase and reabsorbed into the blood from the intestine [7].
Phototherapy is a treatment widely used to reduce serum bilirubin in jaundiced newborns, due to the safety and convenience of its use. As a yellow pigment, bilirubin strongly absorbs blue light in the 460 nm wavelength and then converts it irreversibly to lumirubin, a water-soluble and less toxic compound, which can be excreted with bile acids and urine [2].
The human GIT microbiota contributes to host metabolism. GIT microbiota produces short-chain fatty acids (SCFAs) and secondary bile acids, which play essential roles in host energy metabolism [8]. Primary bile acids, including cholic acid (CA) and chenodeoxycholic acid (CDCA), are synthesized in the liver and then converted to secondary bile acids by microbiota in the GIT [9–11]. Bile acids not only play roles in several metabolism processes, but also regulate the composition of the GIT microbiota [12]. In short, microbial richness is considered to be an indicator of a host’s heath status. Alterations to the microbiota may lead to functional amelioration of the GIT microbiota’s capacity to produce metabolites.
In the present study, the aim was to assess the benefit of treatment for hyperbilirubinemia with phototherapy. In this context, we characterized the 16S rRNA microbiome of jaundiced infants before and after phototherapy, with or without antibiotic treatment. We also quantified the concentrations of secondary bile acids and determined the relationship between the differential microbiota species and differential secondary bile acids. We observed several species/genera and secondary bile acids which may well participate in the clearance of bilirubin in jaundiced infants after they had been treated with phototherapy.
Material and methods
Study cohort and patient characteristics
Fifty-three term neonates with jaundice were treated in the neonatal intensive care unit of the Pediatrics Department, Hospital between June 2017 and June 2018. The whole cohort comprised infants with birth weight ≥ 2,500 g and gestational age over 37 weeks. This study was approved by the ethics committee of our hospital. Written consent was obtained from the legal guardians of these neonates before they were included in the study. All neonates with jaundice were diagnosed according to the criteria defined in practical neonatology. Briefly, neonates with the following symptoms were diagnosed with jaundice. (1) jaundice appears within 24 h after birth; (2) a serum bilirubin concentration > 221 μmol/l (12.9 mg/dl), which was mainly indirect bilirubin, or the daily increase exceeded 85 μmol/l (5 mg/dl); (3) jaundice appears again after receding; (4) jaundice lasted for > 2 weeks and the serum conjugated bilirubin concentration was > 34 μmol/l (2 mg/dl). Exclusion criteria were: congenital malformation; surgical disease; chromosomal abnormalities; congenital metabolic abnormalities; neonatal asphyxia; sepsis or suspected sepsis; RH hemolysis requiring blood exchange; glucose-6-phosphate dehydrogenase (G6PD) deficiency; hemoglobin disease or congenital infection.
Treatment
The blue LED light of the jaundice treatment apparatus with a double-sided light source (peak wavelength 425–475nm) was used. The blue light source was about 40 cm away from the neonates. During phototherapy for 24 h or 48 h the whole body, except for the genitals and eyes, was fully exposed to the light. Breathing and heart rate were closely monitored during the phototherapy treatment.
Determination of serum bilirubin concentration
The routine biochemistry panel, including serum concentrations of bilirubin, was carried out. The fifth-generation Total Bile Acids Randox RX Series assay (Randox Laboratories, Crumlin, UK) was used to measure the total concentrations of bile acids in sera.
Fecal sample collection and storage
In this pilot study, only a subset of 8 samples at baseline, 9 samples after 24 h phototherapy and 12 samples after phototherapy for 48 h were selected for analysis. Samples were selected randomly. Sterile containers were used to collect the fecal samples. Samples were collected on the day of hospital admission, and 48 h and 72 h after phototherapy. Samples were immediately frozen at –20°C and stored until required for subsequent analysis.
Extraction of fecal genomic DNA
Fecal samples were collected in sterile containers and stored at –20°C until required for further analysis. Genomic DNA was purified using a QIAamp PowerFecal DNA Kit according to the instructions of the manufacturer. The libraries were constructed using a standard protocol and the sequencing was produced on the Illumina Hiseq system. Sequence reads were assembled with SOAP de novo. The relative abundance of the microbial species was analyzed using MetaPhlAn.
Quantification of fecal bile acids
The major bile acids (BAs) were identified using gas chromatography. For protein precipitation, each ~100 mg fecal sample was mixed with 0.3 ml of methanol, followed by vortex mixing for 1 min. After 10 min centrifugation (12,000g) at 4°C, the supernatant was transferred and diluted 10 times in methanol, followed by another 1 min of vortex. After a further additional 10 min centrifugation (12,000g) at 4°C, the supernatant (5 μl) was injected into the UPLC (Waters ACQUITY UPLC, US) for analysis.
Chromatographic separation was accomplished in a Thermo Ultimate 3000 system equipped with an ACQUITY UPLC BEH C18 (100 × 2.1 mm, 1.7 μm) Waters column maintained at 40°C. Gradient elution of analytes was carried out with 0.01% formic acid in water (A) and acetonitrile (B). An increasing linear gradient of solvent B (v/v) was used as follows: 0–4 min, 25 % B; 4–9 min, 25–30% B; 9–14 min, 30–36% B; 14–18 min, 36–38% B; 18–24 min, 38–50% B; 24–32 min, 50–75% B; 32–35 min, 75–100% B; 35–38 min, 100–25% B. The capillary temperature was 500°C. The Orbitrap analyzer scanned over a mass range of m/z 81–1000 for a full scan at a mass resolution of 70,000.
Data dependent acquisition (DDA) MS/MS experiments were performed with an HCD scan. The normalized collision energy was 30 eV. Dynamic exclusion was implemented to remove some unnecessary information in the MS/MS spectra.
Ethics approval
The study was approved by the Ethics Committee of Shanghai Sixth People’s Hospital East Campus. Approval No: 2017-009.
Statistical analysis
SPSS 23 was used to analyze the data, which are presented as the mean ± SE. The Wilcoxon rank sum test was employed for comparisons between groups with a non-normal distribution. Correlations between variables were evaluated using the Spearman coefficient. A p-value < 0.05 was considered to be a statistically significant finding.
Results
Clinical characteristics of infants with jaundice
Of the 53 jaundiced infants enrolled in the study, all had been admitted to the NICU of the Sixth People’s Hospital Affiliated to Jiaotong University, Shanghai, between June 2017 and June 2018. The average gestational age was 271.2 ±8.77 days and birth weight 3.2 ±0.36 kg. The onset of jaundice occurred 2.17 ±1.43 days after birth. The mean total serum bilirubin (TSA) was 19.7 ±3.7 mg/dl, and transcutaneous bilirubin (TCB) concentration was 21.49 ±4.36 mg/dl. Twenty-nine of the babies were treated with antibiotics, and the others were not (Table I).
Table I
Baseline and clinical characteristics (n = 40)
Phototherapy effects on total serum bilirubin
The concentration of total serum bilirubin was measured at baseline and after treatment. At the time of hospitalization, the mean serum bilirubin of all the patients combined was 19.7 ±3.7 mg/dl. It was 19.2 ±2.8 mg/dl and 20.1 ±4.3 mg/dl in the non-antibiotic-treated group and antibiotics-treated group, respectively. As shown in Table II, the phototherapy cleared the bilirubin significantly. In the group of non-antibiotic-treated patients, the mean serum bilirubin level decreased to 13.7 ±2.7 mg/dl and 0.8 ±0.2 mg/dl 24 h and 48 h after phototherapy treatment, respectively. In the group of antibiotic-treated infants, it decreased to 13.2 ±3.2 mg/dl and 0.7 ±0.2 mg/dl 24 h and 48 h after phototherapy, respectively. Furthermore, there was no significant difference between the two groups.
Table II
Effects of phototherapy on total serum bilirubin concentrations before and after phototherapy
Phototherapy effects on abundance of microbiota in the GIT
To evaluate the alteration of GIT microbiota before and after phototherapy, Macrogene sequencing analysis was employed. Among the infants who did not receive antibiotic treatment (Figure 1 A), many species detected were significantly altered. Alistipes putredinis and Cellvibrio unclassified were found to be decreased after phototherapy, while Clostridium bolteae, Enterobacter cloacae, Enterococcus faecium, Eubacterium siraeum, Finegoldia magna and Veillonella atypica were found to be increased significantly. Genera including Alistipes, Bacillus, Cellvibrio, Enterobacter, Finegoldia and Lactococcus were altered significantly after phototherapy. On the other hand, among the infants who were given antibiotic treatment (Figure 1 B), species were also found to have been altered significantly after phototherapy. Many species increased including Bacteroides caccae, Dorea unclassified, Eubacterium rectale, Faecalibacterium prausnitzii, Roseburia unclassified, Veillonella parvula, and Veillonella unclassified. Only Pantoea unclassified was found to be decreased after phototherapy. The genera included Clostridium, Eubacterium, Faecalibacterium, Pantoea, Prevotella, Roseburia and Veillonella. These results indicated that phototherapy could modulate the relative abundance of some species and genera. It is noteworthy that the differential species or genera were quite different in infants who were treated with antibiotics and those who were not.
Phototherapy effects on relative abundance of BAs
The microbiota metabolizes the primary bile acids to secondary BAs. LC/MS was used to evaluate the fecal BA profiles in the infants enrolled in the study. Figure 2 demonstrates that phototherapy could induce alterations in some types of secondary bile acids, as indicated by analysis of the fecal samples. In the group of non-antibiotic-treated infants (Figure 2), the concentrations of 11 secondary bile acids were significantly altered after phototherapy, including LCA, 12-ketol LCA, β-UDCA, DCA, NorCA, GLCA, GUDCA, GDCA, TLCA, THDCA and TUDCA. All differential secondary bile acids were elevated 24 h after phototherapy and then reduced to a lower level 48 h after phototherapy, as compared with baseline. On the other hand, in the group of antibiotic-treated infants (Figure 3), levels of 8 secondary bile acids were altered after phototherapy, i.e. LCA, isoLCA, NorCA, GLCA, GUDCA, GDCA, LCA-3S and TLCA. In detail, levels of LCA and LCA-3S were reduced 24 h or 48 h after phototherapy compared to baseline. isoLCA, Nor-CA, GLCA, GUDCA, GDCA and TLCA were elevated 24 h after phototherapy and then were reduced to lower concentrations 48 h after phototherapy compared to baseline values. These results strongly suggested that phototherapy could affect the production of bile acids.
Impact of differential species or genera on differential bile acids
To explore further the association between the differential species/genera and the differential secondary bile acids, multivariate logistic regression analyses were conducted. First, we assessed the effect of differential species or genera on bile acids’ alteration when adjusting for the use of probiotics or breastfeeding. Notably, among the infants who did not receive antibiotic treatment (Figure 4 A), the alteration of the genus Bacillus was associated with β-UDCA (OR: 1.2115 × 1017, 95% CI: 1.5561 × 109 –9.4322 × 1024). Among the infants given antibiotics (Figure 4 B), the alteration of LCA was associated with Bacteroides caccae (OR = 0.0033, 95% CI: 0.0003–0.0342), Veillonella parvula (OR = 66122791.02, 95% CI: 134159.7–32589693406), Veillonella unclassified (OR = 2.4282 × 10–8, 95% CI: 5.3115 × 10–11–1.1100 × 10–5) and the genus Veillonella (OR = 0, 95% CI: 0–6.3239 × 10–271). The alteration of LCA-3S was associated with Bacteroides caccae (OR = 1.6320 × 10–5, 95% CI: 2.9602 × 10–6–8.9978 × 10–5), Roseburia unclassified (OR = 0, 95% CI: 0–0), Veillonella parvula (OR = 145,925.7967, 95% CI: 1,569.550155–13,567,160.04), Veillonella unclassified (OR = 0.000547188, 95% CI: 6.2182 × 10–6–0.04815). The alteration of GDCA was associated with Pantoea unclassified (OR = 1.3752, 95% CI: 1.2344–1.5320).
Figure 4
Differential gut microbial species/genera impact on secondary bile acids. A – Heatmap of odds ratio between differential gut microbial and differential secondary bile acids in infants without antibiotic treatment. B – Heatmap of odds ratio between differential gut microbial and differential secondary bile acids in infants with antibiotic treatment. Rows represent differential microbial species/genera and columns represent differential secondary bile acids
*p < 0.05, **p < 0.01, ***p < 0.001.
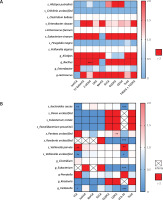
Impact of differential bile acids on differential species or genera
Next, we assessed the effect of differential bile acids on differential species or genera after adjusting the data for the use of probiotics or breast-feeding. Notably, among the infants who were not treated with antibiotics (Figure 5 A), the alteration of the species Eubacterium siraeum was associated with 12-ketoLCA (OR = 1.0233, 95% CI: 1.0017–1.0453), DCA (OR = 1.0074, 95% CI: 1.0012–1.0136) and GLCA (OR = 0.7460, 95% CI: 0.5785–0.9619, p < 0.05). The alteration of the genus Bacillus was associated with β-UDCA (OR = 1.0088, 95% CI: 1.0030–1.0146), DCA (OR = 1.0043, 95% CI: 1.0015–1.0071) and GDCA (OR = 0.917, 95% CI: 0.8680–0.9692). On the other hand, alteration of the genus Lactococcus was associated with GLCA alteration (OR = 0.1440 95% CI: 0.0318–0.6512). In contrast, among the infants given antibiotics (Figure 5 B), the species Bacteroides caccae was associated with isoLCA (OR = 3.2794 × 1016, 95% CI: 5.4541 × 1013–1.9718 × 1019) and GLCA (OR = 2.6089 × 10-64, 95% CI: 2.6234 × 10109–2.5944 × 10-19). Also, the species Eubacterium rectale (OR = 1.6634, 95% CI: 1.2759–2.1685) and Faecalibacterium prausnitzii (OR = 1.5533, 95% CI: 1.2476–1.9340) and the genera Eubacterium (OR = 1.6642, 95% CI: 1.2755–2.1712), Faecalibacterium (OR = 1.5533, 95% CI: 1.2476–1.9340), and Prevotella (OR = 1.6330, 95% CI: 1.2486–2.1357) were associated with alterations in TLCA.
Figure 5
Differential secondary bile acids’ impact on gut microbial species/genera. A – Heatmap of odds ratio between differential gut microbial and differential secondary bile acids in infants without antibiotic treatment. B – Heatmap of odds ratio between differential gut microbial and differential secondary bile acids in infants with antibiotic treatment. Rows represent differential microbial species/genera and columns represent differential secondary bile acids
*p < 0.05, **p < 0.01, ***p < 0.001.
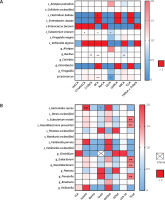
Discussion
The neonatal intestinal microbiota was influenced by several factors including diet, the maternal microbiome and local culture, which may have produced geographical differences in the microbiota composition in the cohort of infants [13]. However, neonatal jaundice is a common clinical sign in newborn infants worldwide, for which phototherapy is the simplest treatment. Hence, it will be necessary to determine the GIT microbiota composition in jaundiced infants before and after phototherapy in different regional-based cohorts. Antibiotics are widely used in neonatal medication, but they perturb a developing infant’s intestinal microbiota. In the present study, infants treated with antibiotics or not had quite a different differential species/genera before and after phototherapy, which supports the disruption effect of antibiotic therapy. More importantly, various factors, including antibiotic-resistant genes, should be taken into consideration when trying to understand the responses of infants to antibiotics.
Phototherapy could transform the unconjugated bilirubin into conjugated forms, which could then be metabolized to urobilinoids by the GIT microbiota. In our study, the TSB of the jaundiced infants dropped drastically in the first 24 h after phototherapy, and then returned to normal values 48 h after phototherapy. By contrast, the relative abundance of fecal secondary bile acids rose rapidly in the first 24 h after phototherapy, but decreased to the level of baseline in the second 24 h. Accordingly, the correlated microbiota species also exhibited a higher relative abundance 24 h after phototherapy compared to baseline and then a reduction 48 h after phototherapy. This finding suggested that TSB reduction in the first 24 h is sufficient to induce the alteration of differential species/genera and secondary bile acids. The underlying mechanisms of this response remain to be investigated.
Reduction of enterohepatic circulation by accelerated bilirubin fecal excretion can reduce infant jaundice. It is well known that exposure to phototherapy provokes an increased rate of intestinal transit and thus diarrhea in jaundiced infants [14, 15]. It is worth thinking about how phototherapy can induce an alteration in the GIT microbiota species. Previous studies have indicated a significantly greater colon motility index for patients with diarrhea, as excess bile acid concentrations in the colon stimulate enteroendocrine cells and accelerate colonic transit. In our study, we observed elevation of fecal secondary bile acids after phototherapy, which leads to speculation that bilirubin metabolism after phototherapy might modulate colonic microbiota species and thus produce more fecal secondary bile acids accordingly. There is limited information on the mechanisms involved in the colon motility response to secondary bile acids. However, the bile acid-GIT microbiome axis has emerged as an important regulator of health and findings have indicated that species interacted with each other. Indeed, we find relationships between differential secondary bile acids and differential species/genera. TLCA (taurolithocholic acid) and DCA are activators of Gαs-protein-coupled receptor G protein-coupled bile acid receptor1 (TGR5), whose activation protects the intestinal barrier function and reduces inflammation [16]. We found that changes in TLCA composition had an impact on several differential species alterations after phototherapy. Among them, Eubacterium rectale, Faecalibacterium prausnitzii, and the genus Prevotella, which are SCFA-producing bacteria [17, 18], were affected by TLCA. Several studies have reported that fecal DCA could be increased by a western diet and participate in intestinal carcinogenesis [19]. Nevertheless, carcinogenesis is a long-term process with stepwise accumulation of mutations. Therefore, transient elevation of DCA after phototherapy might be a stress reaction, and how it modulates GIT homeostasis after phototherapy remains to be explored.
In conclusion, the present study revealed fluxes in bile acids and their association with differential species/genera before and after phototherapy treatment. We believe that a large-cohort study needs to be conducted to confirm the physiological roles of these differential species and BAs.