Introduction
Coronary artery disease (CAD) accounts for approximately 610,000 deaths annually, being the third leading cause of mortality worldwide [1]. CAD is a complex process characterized by the development of atherosclerotic plaques that, when they undergo rupture, erosion, hemorrhage, thrombosis or cause lumen stenosis, lead to acute coronary syndromes (ACS) [2]. ACS is increasingly recognized as a mixture of two pathophysiological phenotypes, namely, a ruptured fibrous cap and a plaque erosion with an intact fibrous cap. Both characteristics lead to thrombus formation and coronary occlusion [3]. However, it is worth recalling that 52.3% of sudden cardiac deaths due to CAD cannot be explained by acute plaque complications [4]. Thus, the volume and composition of the plaque, within the coronary tree, play a critical role in the prognosis of patients with CAD [5]. Indeed, as recently demonstrated, atherosclerotic cardiovascular disease risk parallels the amount of atherosclerosis, regardless of stenosis or prior revascularization procedures [6]. Overall, given that it is plaque instability, not reduced perfusion, which causes myocardial infarction (MI), the question arises why ischemia testing should be superior to an anatomic test. Indeed, it is believed that although ischemia and CAD severity are correlated and inextricably linked, ischemic burden is an imprecise and insensitive surrogate marker of coronary plaque burden [7]. Thus, the aim of this narrative review is two-fold: first, to discuss the current knowledge on plaque and ischemic burdens; and second, to describe the available imaging techniques to define the changing paradigm in the management of atherosclerotic CAD in clinical practice. To pursue this goal, using pubmed.gov, the following algorithm was used: coronary artery disease or acute coronary syndrome or coronary plaque burden or ischemia and coronary artery bypass grafting and coronary artery calcium and coronary computed tomography angiography and cardiac magnetic resonance and computed tomography and computed tomography perfusion and fractional flow reserve and late gadolinium enhancement and myocardial perfusion imaging and optical coherence tomography and positron emission tomography and stress echocardiography and single-photon emission computed tomography. Relative to clinical studies, the search for literature comprised observational, prospective and interventional studies. GS and AF screened the titles and full text of papers identified in our search.
From the ischemia to the plaque concept
Nowadays, major adverse cardiac events (MACE) seem to be related to complex and heterogeneous plaques. They can be obstructive, non-obstructive, can contain regions of flow-limiting or no-flow-limiting obstructions [8]. Thus, a paradigm shift from ischemia to the plaque concept is required as a consequence of clinical-based evidence.
An analysis of the COURAGE (Clinical Outcomes Utilizing Revascularization and Aggressive Drug Evaluation) trial, evaluating the impact of the anatomical and ischemic burden of obstructive disease, showed that in patients undergoing baseline angiograms and single-photon emission computed tomography (SPECT), the degree of ischemia did not predict the clinical outcomes. Conversely, death, MI, and non-ST-segment elevation ACS were predicted by the extent of the anatomical involvement of coronary arteries [9]. The presence of baseline left ventricular (LV) dysfunction and the anatomical burden of atherosclerotic disease were predictors of the combined endpoints of death, MI or acute non-ST-segment elevation coronary syndromes. According to these results, most of the clinical events are given by the presence and the extent of a vulnerable plaque (e.g., a non-obstructive plaque culminating in a sudden occlusion of a previously functioning conduit). The main findings of COURAGE appear the same as those of the ISCHEMIA (International Study of Comparative Health Effectiveness with Medical and Invasive Approaches) study [10]. Overall, there was no prognostic benefit of performing revascularization in patients with stable CAD and of moderate to severe myocardial ischemia. The BARI 2D (Bypass Angioplasty Revascularization Investigation 2 Diabetes) [11] and COURAGE [12] studies failed to demonstrate the superiority of coronary revascularization compared to optimal medical treatment in the occurrence of all-cause death or cardiovascular outcomes in patients with angiographic evidence of obstructive CAD. In a sub-analysis of the ISCHEMIA study [13], it was demonstrated that, irrespective of the ischemic burden, the extent and severity of plaque burden were strong predictors of outcomes across nearly all clinical end points (i.e., all-cause death, cardiovascular death, and MI). In the SCOT-HEART (Scottish Computed Tomography of the Heart) [14] and PROMISE (Prospective Multicenter Imaging Study for Evaluation of Chest Pain) [15] studies, the superiority of anatomic assessment (coronary computed tomography – CT) over functional ischemia testing (exercise electrocardiography, nuclear stress testing, or stress echocardiography) was demonstrated. The PROSPECT study showed that a greater number of vulnerable features (plaque burden of ≥ 70%, minimal luminal area ≤ 4.0 mm2 and virtual histology-defined thin-cap fibroatheroma) was associated with a greater rate of non-culprit lesion-related MACE at a median follow-up of 3.4 years [16]. It should not be overlooked that non-obstructive plaques are often not identified during stress testing. In 543 patients with chest pain or multiple risk factors for CAD, who underwent coronary computed tomography angiography (CCTA), at least one of the vulnerable features was detected in 274 plaques in 182 patients, despite a normal exercise-stress myocardial perfusion SPECT [17]. Moreover, the CREDENCE (Computed Tomographic Evaluation of Atherosclerotic Determinants of Myocardial Ischemia) trial demonstrated that a comprehensive anatomic interpretation with coronary CT, including quantification of obstructive and non-obstructive atherosclerotic plaques, was superior to functional imaging in the diagnosis of invasive fractional flow reserve (FFR). Thus, comprehensive coronary CT measures could improve the prediction of vessel-specific coronary physiology in a more accurate way than stress-induced abnormalities in myocardial perfusion imaging [18]. The 3V FFR-FRIENDS (Clinical Implication of 3-vessel Fractional Flow Reserve) study evaluated the association between physiological disease burden per vessel (FFR) and quantitative and qualitative plaque characteristics (CCTA) and their prognostic implications. The number of high-risk plaque features increased with decreasing FFR with a significant association with the cumulative incidence of the composite vessel-oriented outcome. This study concluded that a better prognostic stratification of patients is obtained by integrating stenosis severity and plaque vulnerability, especially in patients with an FFR > 0.80 [19]. Similar conclusions were reached in diabetic patients. Despite the absence of inducible ischemia, 25% had plaques with thin-cap fibroatheroma associated with a five-fold higher rate of MACE [20]. These results strengthen the assumption that relevant atherosclerotic burden and vulnerable plaques are present despite the absence of ischemia, denoting an increased cardiac risk. Combining information relative to concomitant obstructive and non-obstructive lesions could help stratifying patients’ risk and guiding a more tailored treatment. The main studies regarding inducible ischemia and plaque burden are reported in Table I.
Table I
Main studies about ischemia and plaque
First author | Inclusion criteria | Methods | Outcomes |
---|---|---|---|
Mortensen et al. [5] | Symptoms suggestive of CAD | Follow-up after CCTA examination and CACS | Total coronary atherosclerotic plaque burden (not stenoses per se) is the main predictor of future CVD events and death |
Ibanez B et al. [93] | Middle-aged asymptomatic males and females | Primary prevention screening comprehensive of arterial US and CCTA | Assessment of carotid and femoral plaque burden with ultrasound may be considered a risk modifier in absence of CACS |
Budoff MJ et al. [41] | Patients with stable chest pain (or dyspnea) and intermediate pre-test probability for CAD | Functional testing (exercise electrocardiography, nuclear stress, or stress echocardiography) vs. anatomic testing (CCTA) | Adverse events occurred in > 50% of patients with normal functional testing, while CAC score = 0 is associated with a very low event rate |
Frye RL et al. [11] | Type II diabetic patients with CAD | Prompt revascularization (PCI or CABG) together with OMT vs. OMT alone | No significant difference in the rates of death and major CVD events between groups |
Boden WE et al. [12] | Stable CAD and evidence of myocardial ischemia | PCI with OMT vs. OMT alone | No significant difference in the rates of death and major CVD events between groups |
Mancini GBJ et al. [9] | Stable CAD patients treated with OMT with or without elective or symptom-warranted PCI | Assessment of baseline ischemic burden with quantitative SPECT and anatomical burden with quantitative coronary angiography | Anatomic burden of coronary disease, but not ischemic burden, predicted the risk of death, MI, and NSTE-ACS |
Maron DJ et al. [10] | Stable CAD and moderate or severe reversible ischemia | PCI with OMT vs. OMT alone with a median of 3 years of follow-up | No prognostic benefit of PCI in stable CAD and moderate to severe myocardial ischemia |
Reynolds HR et al. [13] | Stable CAD and moderate or severe reversible ischemia | Investigation of severity of CAD through CCTA and ischemia in PCI with OMT vs. OMT alone | Ischemia severity was not associated with increased risk after adjustment for CAD severity |
Williams MC et al. [14] | Symptomatic patients with suspected CAD | Assessment of prognostic implications of adverse coronary plaque characteristics evaluated by CCTA | Adverse coronary plaque characteristics and CACS confer an increased risk of CVD events |
Douglas PS et al. [15] | Symptomatic patients with suspected CAD | CCTA vs. functional stress testing | CCTA was associated with lower incidence of negative-result invasive catheterizations |
Otsuka et al. [17] | Symptomatic CAD or multiple risk factors patients with normal functional stress testing | CCTA evaluation including CACS and plaque morphology assessment | Physiologically non-obstructive but vulnerable coronary plaques are associated with future ACS events |
Liu T et al. [94] | Patients with suspected CAD | CCTA and stress/rest SPECT-MPI evaluation | Vulnerable plaque characteristics detected 33% patients despite a normal exercise stress test |
Stuijfzand WJ et al. [18] | Symptomatic CAD patients referred to nonemergent invasive coronary angiography | CCTA and stress myocardial perfusion imaging followed by ICA with FFR measurements | CCTA assessment improves prediction of vessel-specific coronary physiology more than stress-induced tests |
Lee JM et al. [19] | Patients with > 30% coronary stenosis | Evaluation of both CCTA and FFR | FFR severity and the number of high-risk plaques are closely related and both associated with significant risk of clinical events |
Kedhi et al. [20] | Diabetes mellitus patients with fractional flow reserve (FFR)-negative lesions | Evaluation of coronary plaques by optical coherence tomography (OCT) and FFR assessment | Plaques with thin-cap fibroatheroma are associated with a 5-fold increased rate of MACE despite the absence of ischemia |
[i] CABG – coronary artery bypass grafting, CAD – coronary artery disease, CACS – coronary artery calcium score, CCTA – coronary computed tomography angiography, CVD – cardiovascular disease, FFR – fractional flow reserve, ICA – invasive coronary angiography, NSTE-ACS – non-ST-elevation acute coronary syndrome, OMT – optimal medical therapy, PCI – percutaneous coronary intervention, US – ultrasound, SPECT – single-photon emission computed tomography, SPECT-MPI – single-photon emission computed tomography myocardial perfusion imaging.
Multimodality imaging for ischemia research
The European guidelines for the diagnosis and management of chronic coronary syndromes [21] recommend the use of either anatomical or non-invasive functional imaging as an initial test for the diagnosis of CAD after the clinical risk assessment. While anatomical imaging describes coronary anatomy with visual identification of stenosis and description of plaques features, functional imaging identifies myocardial ischemia in a coronary territory (Table II). The integration between anatomical and functional features are the goal of multimodality imaging for CAD assessment.
Table II
Characteristics of the main imaging methodologies to assess ischemia
Coronary computed tomography angiography
Coronary computed tomography angiography has shown great technological improvements over the last decades, as current CT scanners ensure high quality images, with reduced contrast volume and radiation dosage. CCTA is the recommended imaging technique in symptomatic patients with a low-intermediate pre-test probability of CAD [22]. The results of the EVINCI (Evaluation of Integrated Cardiac Imaging in Ischemic Heart Disease) study, which evaluated CCTA and several tests (including nuclear imaging, echocardiography, and cardiac magnetic resonance (CMR)), in patients with stable chest pain, concluded that CCTA was the most accurate non-invasive imaging modality for the detection of significant CAD [23]. The PROMISE [24] and the SCOT-HEART [25] studies demonstrated the effectiveness of CCTA as an instrument for prediction of cardiovascular events compared to functional tests and standard care in the setting of stable chest pain. CCTA can also be employed to calculate a single score comprising the total atherosclerotic burden (e.g., the Leiden CCTA risk score). This score provides different weights for coronary plaque presence, extent, severity, composition, and location. Data from a large multi-center CCTA registry showed that the Leiden CCTA risk score was independently associated with MACE, although influenced by sex and age. Women developed coronary atherosclerosis approximately 12 years later than men. Post-menopausal women within the highest atherosclerotic burden group were at significantly higher risk for MACE than their male counterparts [26]. These results establish a connection between the accelerated development of atherosclerosis and a heightened risk for women, despite similar levels of atherosclerotic disease in both genders. Several factors explain this phenomenon. Firstly, estrogen in pre-menopausal women protects against atherosclerosis by improving serum lipid profiles and by causing vasodilatory effects on the blood vessels. It also prevents changes associated with vascular injury and damage to endothelial cells [27]. A decrease in these protective actions can lead to the progression of arterial plaque, which might further cause the plaque to destabilize and lead to acute coronary syndrome. Additionally, women may experience more severe impacts on coronary flow than men for the same level of atherosclerotic disease due to their smaller luminal volume of the 17-segment coronary tree [28]. This could mean more potential heart damage in the future. Furthermore, factors such as reduced collateral blood flow, lower coronary flow reserve, and increased vascular stiffness in women could also play a role.
Emerging applications of CCTA allow non-invasive assessment of the functional significance of atherosclerotic lesions [29]: FFR-CT and CT perfusion can improve the understanding of the hemodynamic significance of plaques. FFR-CT is a technology whereby patient-specific models of blood flow are constructed from CCTA images and used to noninvasively estimate FFR. It creates a patient-specific physiologic model based on computational fluid dynamics and image-based modelling that allows the determination of rest and hyperemic coronary flow and pressure from CCTA scans [30] and is validated against optical coherence tomography (OCT) [31].
When the diagnostic performance of anatomical and functional CCTA for the detection of hemodynamically significant CAD is compared to invasive FFR, the specificity of FFR-CT and its combined use with CCTA is higher (78% and 80%, respectively) than CCTA alone (61%) [32]. Data from the ADVANCE (Assessing Diagnostic Value of Non-invasive FFRCT in Coronary Care) registry concluded that in stable symptomatic patients diagnosed with CAD on CCTA, there was no death or MI within 90 days in those with an FFR-CT > 0.80 [33].
Stress myocardial computed tomography perfusion (CTP) imaging is a CT-based examination that combines the information provided by anatomy and perfusion. CTP is based on the use of vasodilator stress to induce hyperemia to visualize hypo-perfused myocardium. The diagnostic accuracy of CTP is comparable to that of CMR and PET, with a sensitivity and specificity of 93% and 82%, respectively [34]. Moreover, combining CTP and CCTA significantly improves specificity up to 86%, thus increasing the risk stratification of patients with coronary stenosis [32]. Finally, compared to coronary CCTA and CT-FFR, CTP alone retains the highest prognostic value for MACE [35].
The PERFECTION (PERfusion Versus Fractional Flow Reserve CT Derived In Suspected CoroNary) study concluded that the diagnostic performance of CCTA plus CTP and of CCTA plus FFR-CT is similar [36].
In addition to quantifying coronary stenoses, CCTA has the capability to characterize coronary atherosclerosis with the visual identification and discrimination of high-risk plaques that correlate with adverse prognostic features [37]. As shown in Figure 1, the plaque vulnerability is characterized by the following features, which have prognostic implications [38]: (i) a positive remodeling (an outer vessel diameter 10% greater than the mean diameter of the segments immediately proximal and distal to the plaque); (ii) low-attenuation plaque (a focal central area of plaque with an attenuation density of < 30 Hounsfield units); (iii) spotty calcification (a focal calcification within the coronary artery wall that measures < 3 mm in maximum diameter); (iv) napkin-ring sign (a central area of low-attenuation plaque with a peripheral rim of high attenuation) [14].
Figure 1
Features associated with plaque vulnerability assessed by coronary computed tomography (CT). From top to bottom the pictures show (A) a positive remodeling: the arrow indicates the classical outward plaque expansion; (B) a low attenuation plaque: the arrow indicates the plaque with a CT attenuation of 5 HU (Hounsfield units), thus a lipid-rich one (a CT attenuation value of < 30 HU indicates a lipid-rich plaque); (C) a spotty calcification: calcium deposits with a size of < 3 mm are indicated by the arrow; (D) a napkin-ring sign: the attenuation region, surrounded by a higher-attenuation ring (pointed by arrow), is characterized by a necrotic core surrounded by a fibrous cap
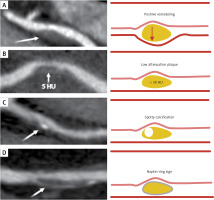
Together with the identification of high-risk plaque features, CT facilitates the detection and quantification of calcified, non-calcified and partially calcified plaque (examples are depicted in Figures 2, 3) [39]. Indeed, CT evaluates atherosclerotic burden in primary prevention through coronary artery calcium (CAC) plaque quantification, which is calculated using the Agatston, volume or mass CAC score [40]. Budoff et al. [41] concluded that patients with CAC scores > 300 are at an equivalent risk of MACE and its components as those treated for established atherosclerotic cardiovascular disease. This observation has important implications related to the therapeutic objectives of cardiovascular prevention in subjects without previous atherosclerotic cardiovascular disease and with high CAC. The PROMISE study showed that in patients with stable chest pain (or dyspnea) and intermediate pre-test probability for obstructive coronary artery disease, more than 50% of adverse events occurred in those with normal stress test (i.e., no exercise- or pharmacologically induced ischemia), in contrast to the measurable CAC score at baseline [42].
Figure 2
Left main coronary artery sub-occlusion in a patient who presented to the emergency department with atypical chest pain. The arrow indicates the sub-occlusive plaque. A, B, D – transversal sections; C – the 3D volume rendering image of the heart
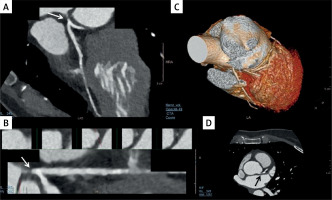
Figure 3
Coronary artery calcifications of the left anterior descending artery (LAD) without critical stenosis in a patient undergoing heart transplant. A – an Agatston score measured in various segments of LAD (mean for LAD 426, total 481). The arrows in panels B and D indicate plaques in medium LAD. C – the 3D volume rendering image of the heart
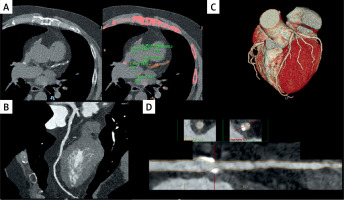
In the context of preventive cardiology, the CAC score is recommended by the American guidelines for lipid management to guide treatment for primary prevention of atherosclerotic CAD in individuals at borderline or intermediate risk [43]. The European guidelines consider the CAC score as a risk modifier to reclassify the risk of cardiovascular diseases in addition to the conventional risk factors. However, caution should be exercised regarding the presence of detected non-calcified plaques [44]. Concerning asymptomatic patients, the CAC score provides an accurate measurement of the coronary calcific plaque burden, as a marker of subclinical atherosclerosis. The MACE rate increases proportionally with increasing severity of coronary calcifications stratified by Agatston calcium score categories 0, 1–99, 100–399, and ≥ 400. Asymptomatic individuals with zero CAC present a persistent very low risk across several studies [45]; recently, the ‘‘power of zero” (the high negative predictive value of a CAC score of zero for the absence of CAD) was extended to the prediction of absent ischemia on positron emission tomography (PET), including in symptomatic patients [46].
Stress echocardiography
Stress echocardiography (SE) is a functional test with wide availability based on the detection of myocardial ischemia, i.e., obstructive coronary atherosclerosis, by observing the transient changes in regional function (reduced decreased wall thickening) that occur during stress [47, 48]. The use of intravenous contrast agents improves endocardial border delineation. In conjunction with wall motion, assessment of coronary flow reserve (CFR), the ratio of hyperemic peak to basal peak diastolic coronary flow Doppler velocities, usually of the left anterior descending artery (LAD), adds potential information [49]. Overall, SE demonstrates very high specificity compared to other functional tests for the detection of obstructive CAD, although it retains a lower sensitivity (possibly improved by CFR) [50]. Overall, in symptomatic patients, the diagnostic accuracy of myocardial perfusion imaging and wall motion imaging is lower compared to CCTA [23]. To improve the diagnostic and prognostic capabilities of SE, the ABCDE protocol was proposed to assess multiple vulnerabilities of ischemic patients. The five steps of the ABCDE protocol are: (A) regional wall motion, (B) B-lines by lung ultrasound assessing extravascular lung water, (C) left ventricular contractile reserve by volumetric two-dimensional echocardiography, (D) coronary flow velocity reserve in the mid-distal left anterior descending coronary with pulsed-wave Doppler; and (E) assessment of heart rate reserve with a one-lead electrocardiogram. Thus, ABCDE stress echo offers insight into five functional reserves: epicardial flow (A); diastolic (B), contractile (C), coronary microcirculatory (D), and chronotropic reserve (E). The ABCDE protocol allows better functional characterization, risk stratification, and personalized tailoring of therapy [51]. However, the comparison with anatomical imaging remains an open question, due to the lack of direct comparative studies. Nevertheless, its prognostic and stratification risk value is well established, as ischemia at SE is an independent predictor of death and hard events [52].
Cardiac magnetic resonance
Stress-CMR can be performed after the injection of a vasodilator drug (adenosine, regadenoson, or dipyridamole). The “coronary steal effect” induces a perfusion deficit that is assessed visually as hypointense areas at the passage of a gadolinium-based contrast agent. If vasodilators are contraindicated, the assessment of myocardial ischemia can be conducted through the infusion of dobutamine with visualization of ventricular wall motion abnormalities. CMR has the uniqueness of permitting tissue characterization with the acquisition of late gadolinium enhancement (LGE) sequences to detect the extent of the infarct scar and consequently assess viability. Indeed, scars not exceeding 25% of myocardial wall thickness are most likely to achieve functional recovery after revascularization, while segments with LGE extension more than 75% are unlikely to recover [53]. When stress-CMR is validated against FFR, the diagnostic ability of CMR perfusion to detect ischemic CAD is high [54]. Furthermore, stress-CMR has similar sensitivity and specificity of CCTA and PET and seems superior to both SPECT and dobutamine SE when FFR is used as a reference standard [55]. In the direct comparison with SPECT, there is a higher spatial resolution, a larger field of view, and better tissue differentiation. Because stress-CMR can identify subendocardial ischemia, it is less susceptible to balanced ischemia (deceptively normal perfusion images in the presence of multivessel ischemia) than SPECT [56]. Furthermore, at the prognostic level, stress-CMR is associated with a higher risk of cardiac death and adverse events [57].
In the near future, the emerging quantitative analysis of myocardial perfusion using stress-CMR could improve diagnostic utility to better differentiate between single- and multi-vessel disease than visual analysis alone. CMR, despite being a valuable tool for assessing various aspects of heart structure and function, does face limitations, particularly regarding the frame rate and the thickness of each slice: (i) The frame rate in CMR is often lower compared to other imaging modalities such as echocardiography. This means that CMR might not effectively capture rapid cardiac movements, which can be a limitation when assessing dynamic heart function during different phases of the cardiac cycle; (ii) CMR typically employs slices compared to other finer-resolution imaging techniques, such as CT scans. This can lead to partial volume effects, where tissues of different types within the same slice may be averaged together, potentially obscuring detailed structures or pathological findings. These limitations can impact the ability of CMR to provide detailed and accurate real-time imaging, especially for diagnosing conditions that involve subtle or rapid changes in heart structure and function. However, ongoing advancements in CMR technology, such as the development of techniques for higher temporal resolution and the use of thinner slices, continue to improve its diagnostic capabilities [58].
Nuclear imaging
Myocardial perfusion imaging (MPI) characterizes myocardial blood flow by detecting low doses of radioactive tracers at rest and after exercise or pharmacologic stress. MPI includes SPECT and PET. SPECT commonly uses technetium-99 m based (8 to 10 mSV radiation exposure) or less frequently, thallium-201 tracers (18–20 mSV radiation exposure) as single isotopes. PET uses N-13-ammonia or rubidium-82 tracers and 18F-fludeoxyglucose (FDG) [56].
Single-photon emission computed tomography has low spatial resolution and reduced sensitivity for detecting diffuse ischemia due to left main disease or 3-vessel disease (balanced ischemia) [59]. Berman et al. [60] evaluated the effectiveness of SPECT MPI for diagnosing left main CAD in 101 patients who had significant left main CAD (≥ 50% stenosis), without prior myocardial infarction or coronary revascularization. The patients were assessed using technetium 99m sestamibi during stress induced by exercise or adenosine. The study’s findings indicated that (i) perfusion data alone identified high-risk disease (moderate to severe defects affecting over 10% of the myocardium during stress) in only 56% of patients visually and 59% quantitatively; (ii) between 13% and 15% of the patients exhibited no significant perfusion defects (affecting less than 5% of the myocardium); (iii) when analysis included both visual perfusion data and nonperfusion variables, such as transient ischemic dilation, the detection of high-risk patients increased to 83%.
Positron emission tomography assesses both perfusion and metabolism function and can uniquely quantify blood flow, thus allowing detection of multivessel disease as well as the assessment of microvascular dysfunction.
The analysis of metabolism identifies hibernated myocardium in the presence of enhanced FDG uptake in regions with reduced blood flow (“mismatch”), necrotic myocardium when both metabolism and flow show a consistent decrease (“match”), and stunning myocardium when there is decreased metabolism but normal perfusion. The main limitations of PET are the availability and elevated costs [61].
All in all, although the most recent evidence demonstrates the higher diagnostic power of anatomical imaging, the European guidelines [21] recommend that in patients with an intermediate-high clinical likelihood of CAD, a functional ischemia test should be preferred before coronary angiography.
Patient follow-up after revascularization: monitoring and addressing symptoms
Patients with chronic coronary syndrome (CCS) are individuals at a high risk of future MACE regardless of whether they have undergone coronary revascularization or not [62]. Both American [63] and European [21] guidelines highlight the need for regular outpatient monitoring, emphasizing the lifelong surveillance of these patients. The key determinant guiding clinical management, follow-up, and the potential use of diagnostic tests hinges on the patients’ clinical stability, primarily determined by the presence of symptoms. This is crucial as the clinical approach varies between these two clinical phenotypes.
Follow-up plan and testing in stable asymptomatic patients
Over the past two decades, there has been a decline in rates of MACE among patients with CCS [64], especially if they adhere to medical therapy, particularly when they are free from anginal symptoms [65]. In stable patients without anginal symptoms and no significant changes in clinical functional status, the clinical approach differs depending on the lag with any prior acute coronary event or coronary revascularization [21]. Patients with stabilized symptoms within 1 year after an ACS event or those who have undergone revascularization should receive more vigilant monitoring since they face a higher risk of complications and may require changes in pharmacological treatment [66]. Specifically, at least two clinical outpatient visits during the first year of follow-up are strongly recommended [21]. For those who have experienced LV systolic dysfunction, either before the revascularization procedure or after the acute coronary syndrome, a reassessment of LV function should be considered within 8 to 12 weeks [67]. Conversely, in stabilized patients more than 1 year after the initial diagnosis or revascularization, an annual evaluation by a cardiovascular practitioner is necessary, even if they are asymptomatic [21]. Periodic recording of a standard resting 12-lead ECG may establish a baseline waveform against which future tracings taken during symptoms can be reasonably compared, helping to avoid overdiagnosis of changes in clinical status [63]. A complete lipid profile, renal function assessment, complete blood count, and potentially biomarker testing, should be conducted every 2 years [66].
As for the use of routine diagnostic tests in stable and asymptomatic patients, international guidelines significantly differ. The European approach suggests a re-evaluation of LV function every 3–5 years through an echocardiogram to assesses also valvular status and cardiac dimensions [21]. In the event that an unexplained reduction in systolic LV function is found, imaging of coronary artery anatomy is consequently recommended [68]. The American guidelines [63], especially considering the results of a post-hoc analysis of the MASS II (the second Medical, Angioplasty, or Surgery Study), suggest a routine reassessment of LV function in asymptomatic patients not presenting with changes in functional status or requiring a clinical intervention. The MASS II study demonstrated that regardless of the therapeutic approach used, LV function remained stable over long-term follow-up in the absence of MACE [69]. While the European guidelines [21] suggest an assessment in apparently asymptomatic patients for silent ischemia every 3–5 years, preferably using stress imaging, conversely, the American guidelines for CCS management strongly emphasize that routinely conducting anatomical or ischemic testing in asymptomatic, non-sedentary patients is not recommended, since there is no impact on hard outcomes [63]. This recommendation is supported by the findings of the large pragmatic POST-PCI (Pragmatic Trial Comparing Symptom-Oriented Versus Routine Stress Testing in High-Risk Patients Undergoing Percutaneous Coronary Intervention) trial [70]. This study compared an active follow-up strategy of routine functional testing after 12 months from revascularization with a standard-care strategy in 1706 high-risk patients who had complex anatomical features (such as multivessel disease) or clinical characteristics (such as diabetes). Within 2 years, the incidence of a composite of death from any cause, MI, or hospitalization for unstable angina did not differ significantly between the two strategies. The routine stress-tested group had more frequent invasive coronary angiography and repeated revascularization after 1 year with no significant reduction in major cardiovascular events or mortality [71]. These findings shed light on the potential limitations and futility of routine surveillance with stress testing in post-PCI patients. This aspect further emphasizes the central role of symptoms when evaluating stable patients after revascularization. While for symptomatic patients it is reasonable to undergo stress testing to exclude residual ischemia and assess functional status, in asymptomatic patients a less aggressive follow-up is plausible. Indeed, routine surveillance for detection of inducible ischemia did not provide an additional treatment effect. Finally, it is well established that in patients whose clinical or functional status remains unchanged, there is no need for routine periodic invasive coronary angiography [21, 63]. This approach has been associated with an increased rate of revascularization of non-ischemic intermediate lesions, without any improvement in the rates of subsequent cardiac death or myocardial infarction [72].
Testing in unstable symptomatic patients
In patients with CCS with changes in symptoms or functional capacity, the initial approach should prioritize the best guideline-directed medical approach and defer diagnostic testing until after a suitable period of therapy [21, 63,73]. However, this option is not easy to employ, as patients at very high risk (e.g., secondary prevention) frequently present to the emergency department with angina symptoms. Within this framework, when patients develop new-onset LV dysfunction, clinical heart failure, regional wall motion abnormalities, or have a history of complex or incomplete revascularization and/or experiences severe or deteriorating symptoms, an invasive coronary angiography adjuvated by invasive functional testing is recommended [21, 63,73]. Conversely, in the absence of these clinical characteristics, patients should undergo anatomical or functional imaging testing. The American guidelines on chest pain propose a differentiated approach based on the presence of obstructive CAD (> 50% stenosis) or a previous coronary revascularization [73]. When symptomatic patients have non-obstructive CAD (< 50% stenosis), in the absence of a prior coronary revascularization, the preferred approach should be CCTA [74] followed by the assessment of functional ischemia by CCTA-derived FFR and/or CCTA myocardial perfusion [75]. If not available, stress imaging should be preferred. In cases where a patient with chest pain has a documented history of obstructive CAD (> 50% stenosis) and/or a previous revascularization, both American and European guidelines are in favor of non-invasive ischemia testing by using stress imaging over anatomical testing [21, 63,73]. This approach is primarily due to the well-documented imaging challenges posed by high-calcific lesions and by intracoronary stents when using CCTA [76]. Despite their respective advantages and disadvantages, it is widely recognized that all available methods, including PET/SPECT MPI, CMR imaging, and SE, can effectively identify the presence and the extent of myocardial ischemia, estimate the risk of MACE, and inform clinical decision-making [77]. In this scenario, non-invasive coronary anatomical assessment through CCTA can be of value, especially due to significant technological advancements (e.g., scanner temporal resolution, detector coverage, the development of model-based interactive reconstruction algorithms) [78]. Indeed, for patients with CCS who continue to experience symptoms or functional limitations despite optimal guideline-directed medical therapy, CCTA stands as the gold standard for evaluating the patency of both venous and arterial bypass grafts [21, 63]. Unlike the European guidelines [21], the American ones [63], the SCCT 2021 Expert Consensus [79] recommend considering CCTA for symptomatic patients carrying stents with a diameter exceeding 3 mm. This is valid especially when employing measures to enhance stent imaging accuracy, such as strict heart rate control (targeting < 60 bpm), iterative reconstruction, sharp kernel reconstruction, and mono-energetic reconstructions. It may also be desirable, particularly in experienced centers, to conduct CCTA in symptomatic patients with stents smaller than 3 mm, particularly when these stents are known to have thin struts (< 100 µm) in proximal, non-bifurcation locations [79]. Finally, the exercise treadmill test, which plays a secondary role in guiding management in this clinical context [80], should be limited to evaluating the relationship between symptoms and graded stress testing. This helps to confirm the diagnosis of angina pectoris and to assess the severity of symptom [63].
For clarity, Figure 4 provides an overview of the guidance outlined in both European and American guidelines for the clinical and instrumental follow-up of patients with chronic coronary syndrome and those who have previously undergone coronary revascularization. The clinical approach differs depending on whether patients are stable or experiencing symptoms. For asymptomatic patients, vigilant clinical monitoring, without the need to perform ischemia tests or anatomical assessments, seems to suffice. Conversely, for symptomatic and unstable patients, the clinical scenario of presentation is the primary criterion to determine the most suitable imaging methods.
Figure 4
Follow-up algorithm in patients affected by chronic coronary syndrome. A scheme is proposed according to the presence of symptoms and based on the different international guidelines (AHA, ESC and STSS)
AHA – American Heart Association, ACC – American College of Cardiology, CAD – coronary artery disease, CCTA – coronary computed tomography angiography, ESC – European Society of Cardiology, FFR – fractional flow reserve, STSS – Society of Thoracic Surgeons Score.
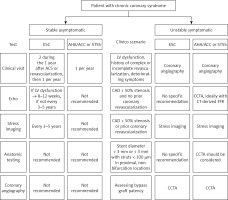
Adherence to lipid-lowering therapies
Although not in the remit of the present review, it is worth mentioning that, in addition to adhering to a diet low in saturated fats and engaging in exercise, lowering low-density lipoprotein cholesterol (LDL-C) with statins as a monotherapy or in combination with ezetimibe reduces plaque progression in patients with CAD [81, 82]. This evidence also applies for monoclonal antibodies against proprotein convertase subtilisin/kexin type 9 (PCSK9) or in the case of eicosapentaenoic acid (EPA) [83]. A meta-analysis of 12 studies examining the effect of statin therapy on different plaque volumes assessed by serial CCTA concluded that intensive statin therapy reduced total plaque volume by 21 mm3, while moderate statin therapy reduced it by 2 mm3. Percentages of mean volume regression were 3.6% and 0.7%, respectively, in intensive and moderate statin recipients. Statins also decreased non-calcified plaque volume by 7.6 mm3 and low attenuation plaque volume by 5.9 mm3. However, calcified plaque volume increased by 11.8 mm3 in the groups given a statin [84]. In line with this evidence, data from the PARADIGM (Progression of AtheRosclerotic PlAque DetermIned by Computed TomoGraphic Angiography Imaging) study, which recruited 613 patients (aged 62.2 years) with mild CAD undergoing serial CCTA at more than a 2-year inter-scan interval, showed that statins reduced plaque progression, particularly in lesions with a higher number of high-risk coronary atherosclerotic plaque features. Key factors for rapid plaque progression included more than 2 high-risk coronary atherosclerotic plaques, diabetes, and smoking [85].
In the HUYGENS (High-Resolution Assessment of Coronary Plaques in a Global Evolocumab Randomized Study) study, which enrolled non-ST-segment elevation ACS patients with interventional treatment of culprit plaque, evolocumab led to plaque stabilization and regression [86]. Similar results were found in the PACMAN-AMI (Vascular Effects of Alirocumab in Acute MI-Patients) study, demonstrating that alirocumab significantly favored coronary plaque regression in non-infarct-related arteries [87].
Although doubts persist regarding the cardiovascular benefit of reducing triglycerides [88], the findings of the EVAPORATE (Effect of Vascepa on Improving Coronary Atherosclerosis in People With High Triglycerides Taking Statin Therapy) study are unequivocal. Among patients with elevated triglyceride and at the maximal dose of tolerated statin, EPA (4 g/day) improved mean distal segment FFR-CT at 9- and 18-month follow-up compared with placebo [89]. In addition to lipid-lowering therapies, patients with residual inflammatory risk have the option of colchicine, which reduces the risk of cardiovascular disease by targeting the inflammatory pathways that influence major cardiac events [90]. The LoDoCo2 (Low-Dose Colchicine-2) study in 2020 provided key data in more than 5000 patients with chronic coronary disease. Colchicine (0.5 mg) reduced cardiovascular death, nonprocedural MI, ischemic stroke, or ischemia-driven coronary revascularization over a median follow-up of 28.6 months compared with placebo [91].
Conclusions and practical guidance
Coronary artery stenosis and its revascularization have been the mainstay of treatment in recent years. Although it maintains a central role in symptomatic patients with obstructive CAD, accumulating evidence demonstrates that atherosclerotic burden may be as important to guide treatment intensity and improve prognosis (see the algorithm we propose in Figure 5). However, while there are clear indications for the timing and intervention of flow-limiting stenosis, management of high atherosclerotic burden or high-risk vulnerable plaques is still debated. Currently, the appropriate management of asymptomatic angina patients and an acceptable quality of life are based on intensive medical therapy. Conversely, the invasive strategy represents the best management for patients with frequent symptoms or angina, features that reduce the quality of life despite intensive medical care therapy. Plaque regression and stabilization may be the most crucial component to ameliorate the atherosclerotic burden and could potentially enhance the magnitude of absolute risk reduction, preventing unwarranted treatment of lower-risk patients. Overall, a method capable of analyzing the coronary anatomy (e.g., CCTA) allows a crucial step to be added in the assessment of patients with suspected or chronic ischemic heart disease. The newly available imaging tools could provide a better insight in choosing the best therapeutic strategy in individuals with high-risk profiles for MACE and identifiable vulnerable plaque morphology and characteristics [92–94].
Figure 5
Proposed algorithm for the risk assessment of cardiovascular disease (CVD) and de novo stratification in apparently healthy asymptomatic patients. The first step consists in the assessment of the 10-year cardiovascular risk by using SCORE2 and SCORE2-OP (in individuals > 70 years old), and in the identification of patients (with age-dependent cut-offs) at low-to-moderate, high, and very high CVD risk. Individuals at low-to-moderate risk do not require further CVD risk stratification through non-invasive imaging approaches. Conversely, individuals at high risk may benefit from the identification of subclinical atherosclerosis through an anatomical approach (e.g., CCTA, CAC and carotid ultrasound). This allows reclassification of the CVD risk towards low-to-moderate or very high risk. Individuals at very high risk may undergo screening for underlying CAD through three different approaches: pure anatomical (CCTA), pure functional (stress-echo, SPECT, and stress-CMR), or combined (stress-CPT and FFR-CT)
CVD – cardiovascular disease, CCTA – coronary computed tomography angiography, CAC – coronary artery calcium, SPECT – single-photon emission computed tomography, CMR – cardiac magnetic resonance, FFR – functional flow reserve.
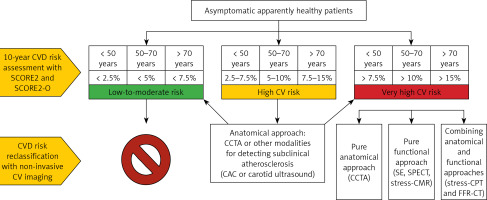