Introduction
Currently, the most common benign tumor, affecting up to 80% of all women of reproductive age, is still uterine leiomyoma (UL) [1]. This pathologic myometrial formation can be a source of considerable quality-of-life issues for approximately 25% of all women who suffer from clinically significant symptoms [2]. The majority of them can be divided into three groups: abnormal bleeding, pressure/pain, and reproductive problems [3]. Moreover, UL has effects on the endometrium and implantation [1]. Until now, risk factors for UL have been described by numerous publications, whereas not one theory exists explaining its pathogenesis. The majority of ULs (60%) are chromosomally normal, and the remainder share similar tumor-specific abnormalities [4]. Undoubtedly, growth factors, cytokines, chemokines and hormonal misbalance play a crucial role in the development of the pathomorphological features of UL (fibrosis, angiogenesis and immune response). The effector cells involved in these processes could be a key opening the nature of widespread gynecological pathology [5]. The following growth factors are among those most involved in the pathogenesis of UL: insulin-like growth factors (IGFs), platelet-derived growth factor (PDGF), vascular endothelial growth factor (VEGF), epidermal growth factor (EGF) and basic fibroblast growth factor (bFGF), heparin-binding epidermal growth factor (HB-EGF), tumor necrosis factor-α (TGF-α), transforming growth factor β (TGF-β), acidic fibroblast growth factor (aFGF) and adrenomedullin (ADM) [6–8]. The impact of sex steroids (estrogen and progesterone) on cell proliferation is well known, while the interaction between them and growth factors is still quite debatable.
Uterine leiomyoma is strongly associated with local hypoxia, which in turn stimulated production of extracellular matrix as well as an angiogenic response in the myometrial tissue [9]. The presence of broad avascular areas within leiomyoma [10] could be an enhancing factor in formation of its own “vascular capsule” at the border between the tumor and the surrounding myometrium [11]. Hypoxia stimulates production of soluble fms-like tyrosine kinase 1 (sFlt-1) or VEGFR-1 (VEGF receptor-1), an anti-angiogenic-related factor. Moreover, it reduces apoptosis in myometrial cells with no further effect on leiomyoma cells [12]. Cells having receptors to PDGF and VEGF and characterized by high sensitivity to hypoxia include telocytes. They also, like hematopoietic stem cells (HSC) [13], have CD34 immunopositivity and are involved in local angiogenesis, as was proved in the cardiovascular system and lungs [14–16].
Telocytes (TCs) are a novel type of interstitial cell population. Telocytes were first described by the Popescu group in 2005 and characterized by a small cell body and extremely long prolongations named telopodes (Tps) with alternating thin segments (podomers) and dilated segments (podoms). Currently, these cells are identified by immunohistochemistry, immunofluorescence and, occasionally, by transmission electron microscopy (TEM). The presence of TCs has been reported in a variety of anatomical units in human and animal bodies. These cells have their own unique morphology, demonstrate specific direct (homocellular and heterocellular junctions) and/or indirect (chemical, paracrine/juxtacrine signaling, microvesicles and exosomes, sex hormones and microRNAs) contact with various surrounding cells, and have gene expression and immunohistochemical profiles [17–22]. Primarily, only eight basic ultrastructural identification criteria were proposed by Huizinga et al. in 1997 and unified as the “gold standard” [23]. Later, the addition of two more precise criteria regarding quantitative estimation of cell organelles and accurate description of cell prolongations by Popescu and his group yielded the “platinum standard” of diagnosis for TCs [20, 21, 24]. The most useful markers for general identification are CD34 and PDGFRα [25, 26].
Dynamics and possible involvement of TCs in pathogenesis were observed in such diseases as systemic sclerosis [27, 28], Crohn’s disease [29], myocardial infarction [30], gallstone disease [18, 31, 32], psoriasis [33], endometriosis and acute salpingitis [34–36], liver fibrosis [37], primary Sjögren’s syndrome [38], keratoconic human cornea [39] and pathologies of the urinary bladder [40, 41]. In addition, a difference in the number of TCs in the myometrium and endometrium during pregnant and nonpregnant states has been described [42–44]. Cretoiu et al. focused on the distribution and role of TCs in the female reproductive system [45, 46].
The interaction of TCs and local angiogenesis in a human leiomyoma has not been clearly verified yet. Several studies have described TCs in UL, while no one has described its interplay with myometrial vascular structure. The aim of our study was to determine the link between myometrial TCs and vascularization in a human leiomyoma, and to clarify their possible role in angiogenesis. We suggest that the interplay of TCs and adjacent cells could reveal new aspects in the pathophysiology of myometrial changes.
Material and methods
Subjects
Twenty patients with symptomatic intramuscular solid UL (mostly located in the fundus of the uterus) were scheduled for elective surgery (laparoscopic hysterectomy) and selected for the study group (20 women, mean age: 56.8 ±10.0 years). The control group consisted of 15 patients (15 women, mean age: 57.6 ±12.7 years) who underwent elective surgery for other reasons and had no pre- or intraoperative signs of uterine fibroids. Hysterectomy was performed according to the standard procedure. Samples of tissue from the foci of fibrosis and adjacent myometrium were taken for further observation from the study group. Samples of unchanged myometrium were also prepared from the control group. All patients were surgically treated at the Institute of Gynecology, Jagiellonian University Medical College in 2018. The study was conducted in accordance with the moral, ethical, regulatory and scientific principles governing clinical research. All surgical samples were retrieved with the approval of the Jagiellonian University Bioethical Committee (protocol number – 122.6120.40.2016) using procedures that conformed to the Declaration of Helsinki guidelines.
Tissue processing
Tissue samples from fresh hysterectomy specimens were collected and rinsed thoroughly with PBS (phosphate-buffered saline, 0.01 M, pH = 7.4), fixed in 4% phosphate-buffered paraformaldehyde, routinely processed and embedded in paraffin. Serial sections were cut and mounted on poly-L-lysine-coated glass slides.
Routine histology
The sections were deparaffinized, rehydrated and stained with either hematoxylin–eosin (H&E) to evaluate the gross tissue organization or Masson’s trichrome staining to detect collagen deposits.
Immunofluorescence
Indirect double immunofluorescence after heat-induced epitope retrieval was used to allow the simultaneous visualization of two antigens. After deparaffinization and rehydration, the slides were incubated for 30 min in PBS with appropriate normal serum at room temperature, followed by overnight incubation at 4°C in a solution of PBS with appropriate normal serum containing primary antibodies. After 5 washes (10 min each) in PBS, the specimens were then incubated for 1 h at room temperature with secondary antibodies diluted in PBS. Finally, the slides were washed in two changes (10 min each) of PBS and cover-slipped with fluorescence mounting medium (Dako, Denmark) and Menzel-Gläser cover glasses. Labeled specimens were analyzed immediately. The primary antisera and secondary antibodies used are listed in Table I.
Table I
Type, sources and dilution of antibodies
Microscopic examination of telocytes, collagen deposits and vascular parameters
Slides were examined using an MN800FL epifluorescence microscope (OptaTech, Warszawa, Poland) equipped with an Olympus DP74 digital CCD camera. Digital images were collected at either 200×, 400× or 600× magnification. The qualitative analysis of cells was provided in 10 consecutive high-power fields of vision (600×) using the computer-based image analysis system Multiscan 18.03 software (CSS, Warszawa, Poland). All samples were assessed by two independent specialists (each blinded to the other) without any knowledge of the clinical parameters or other prognostic factors to avoid bias.
The use of mast cell tryptase staining enabled c-kit-positive mast cells to be distinguished from c-kit-positive TCs. TCs were considered cells that were c-kit positive and tryptase negative concurrently, with the characteristic morphology in tissue samples. Additionally, cells double positive for CD34 and PDGFRα with the characteristic morphology and localization were also recognized as TCs. In order to distinguish populations of CD34-positive cells in the myometrial tissue (vessels and telocytes), we used double immunolabelling for CD34/CD31. Double positive structures were marked as vessels, whereas CD43-positive cells with elongated oval-shape body and long extensions were marked as telocytes. The vascular density was evaluated by the analysis of CD31 and sFlt-1 (VEGFR-1) immunopositivity in all myometrial samples. In all sections the immunoreactive cells found were evaluated with respect to the relative frequency (arbitrarily graded as very few = (+), few = +, moderate density = + +, multiple density = + + +). The percentage of collagen deposits and muscle tissue was analyzed in specimens, stained with Masson trichrome. The collagen and muscle fiber volume ratio was determined in ten consecutive fields of view of each sample.
Results
The histopathological changes in all uterine samples in this study were determined by hematoxylin–eosin and Masson’s trichrome staining. Leiomyoma was composed of interlacing fascicles of uniform spindle cells. The nuclei were elongated, and the cytoplasm was abundant, eosinophilic and fibrillar. The adjacent myometrium and the foci of fibroids were cytologically identical, but the latter was circumscribed and nodular with denser cellularity (Figure 1). Light microscopy of uterine fibroids, adjacent myometrium and normal myometrium using Masson’s trichrome staining for collagen revealed collagen to be abundant in the fibroid tissue, while the myometrium had sparse, well-aligned collagen bundles adjacent to smooth muscle cells. The study group was characterized by the prevalence of collagen deposits compared with the control group. The amount of muscle fibers was lower compared with the adjacent myometrium. Conversely, the percentage of muscle fibers was higher in the normal myometrium, while collagen was scantily present in this type of tissue. In addition, a more significant difference in contents of both components was revealed in the myometrium, surrounding myometrial fibroids. The prevalence of muscle fibers correlates with poor organization of collagen deposits, which was approximately 2.5 times lower compared with muscles (Table II).
Table II
Percentage of collagen and muscle fibers in different types of myometrium
Variable | Affected uterus | Unchanged uterus | |
---|---|---|---|
Fibroid | Adjacent myometrium | Normal myometrium | |
Collagen (%) | 44 ±15 | 18 ±6 | 24 ±10 |
Muscle fibers (%) | 33 ±13 | 45 ±13 | 43 ±10 |
Figure 1
Hematoxylin–eosin and Masson’s trichrome-stained sections of human myometrium. The myometrium sections from the control group (A, C) compared with the foci of leiomyoma (B, D). The myometrium sections from the control group (A) compared with the foci of leiomyoma (B). With Masson’s trichrome staining, collagen deposits were blue in color; muscle fibers, red in color. Fragments of disordered smooth muscle cells were separated by abundant extracellular matrix (ECM). Scale magnification bar: 100 μm
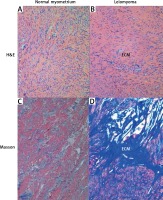
Immunofluorescent labeling was performed for the primary identification of TCs on uterine tissue affected and unchanged by UL. We used current, mostly proven markers, including CD34, PDGFRα and the canonic c-kit. Double immunolabeling for c-kit and tryptase was performed for the identification of mast cells and signs of consequent inflammation. In immunostained slides, c-kit and tryptase double-positive mast cells were generally round or oval shaped, with a centrally located nucleus. The c-kit-positive/mast cell tryptase-negative cells were considered TCs (Figure 2). These cells were mostly fusiform in shape with small branches. They have been detected in UL and adjacent myometrium as well as in normal myometrium from healthy uterus.
Figure 2
Sample of leiomyoma stained for c-kit (red, Alexa Fluor 594) and tryptase (green, Alexa Fluor 488). Double immunopositive cells with round bodies indicated by the bullet arrow are mast cells. The cell with strong immunopositivity only for c-kit and marked by the field arrow is identified as a telocyte. Scale magnification bar: 50 μm
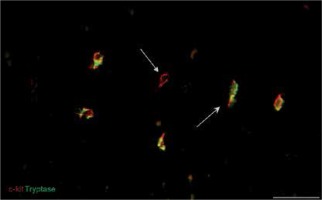
We also found that double immunopositive cells for CD34 and PDGFRα (putative uterine TCs) are present in leiomyomas, adjacent myometrium and normal myometrium. Mostly they had elongated oval-shaped cellular bodies and were distributed among the intertwined myometrial fibers and in close vicinity to blood vessels. The general pattern of their localization resembled parallel eccentric lines. However, in some parts, they reflected directional smooth muscle bundles. CD34/PDGFRα-positive cells were scantily present in the foci of leiomyoma (Figure 3).
Figure 3
Sample of leiomyoma stained for PDGFRa (red, Alexa Fluor 594) and CD34 (green, Alexa Fluor 488). Double immunopositive cells with elongated bodies located between muscle fibers and close to blood vessels are identified as telocytes. Scale magnification bar: 20 μm
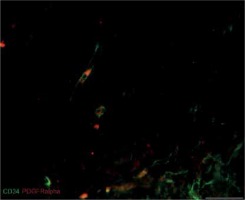
Immunolabeling for CD31 was performed for assessment of vascular density in myometrial tissue from affected and unchanged by leiomyoma samples. The CD31 labeling showed lower expression in the foci of leiomyoma. The normal myometrium as well as that adjacent to the pathology had plenty of CD31-positive cells, which mostly formed circular and longitudinal lines (Figure 4). In addition, double immunostaining for CD34/CD31 revealed cells which were only CD34-positive and had long extensions. They were detected between vessels and myometrial fibers, not rare in close vicinity to small vessels. Their expression was higher in the normal myometrium and lower in that affected by myoma. We assume that CD34-positive cells were uterine telocytes (Figure 5).
Figure 4
Vascular density in unaffected myometrium (A) and leiomyoma (B) , assessed by staining for CD31 (green, Alexa Fluor 488). Strong expression of CD31 cells was common for the healthy myometrial tissue, whereas samples affected by leiomyoma had poor vascularization within the pathological focus. Scale magnification bar: 50 μm
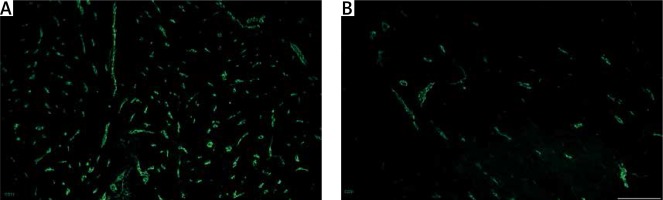
Figure 5
Sample of leiomyoma stained for D31 (red, Alexa Fluor 594) and CD 34 (green, Alexa Fluor 488). Double immunopositive structures were identified as vessels of different caliber, while cells with elongated bodies located between muscle fibers and close to blood vessels were identified as telocytes (one of them is indicated by an arrow in the image). Scale magnification bar: 50 μm
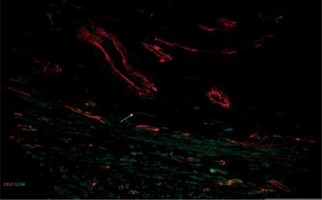
The opposite tendency was observed in samples labeled by VEGFR-1 (sFlt-1). The expression of this marker was higher in the foci of leiomyoma, while normal and adjacent myometrium had fewer sFlt-1 positive cells (Figure 6).
Figure 6
Sample of leiomyoma stained for sFlt-1 (VEGFR-1) (green, Alexa Fluor 488). Immunopositive cells are located mostly close to vessels within leiomyoma; some of them are observed between myometrial fibers. Scale magnification bar: 50 μm
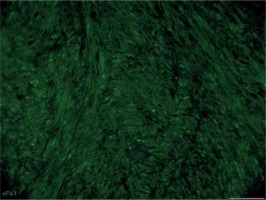
In all sections the immunoreactive cells found were evaluated with respect to the relative frequency. The subjective qualitative analyses showed the decreasing density of TCs in fibroids compared with both types of unchanged myometrium (adjacent and from healthy uterus). The expression of CD31 was higher in the normal myometrium and significantly lower in the foci of leiomyoma. The opposite effect was typical for sFlt-1 expression. Leiomyoma was characterized by increasing of sFlt-1 expression. Results of semiquantitative analysis of CD34 immunoreactivity in TCs from human fetal skeletal muscle samples are summarized in Table III.
Table III
Relative frequency of c-kit-positive/tryptase-negative, CD34–positive and PDGFRα-positive, CD31-positive and sFlt-1-positive cells in different parts of human unchanged uterus and uterus affected by leiomyoma. 0 = absence, (+) = very few, + = few, + + = moderate density, + + + = multiple density
Variable | c-kit+/tryptase- | CD34+/PDGFRα+ | CD31 | VEGFR-1 (sFlt-1) |
---|---|---|---|---|
Normal uterus: | ||||
Unchanged myometrium | +++ | +++ | +++ | + |
Myomatous uterus: | ||||
Adjacent myometrium | ++ | ++ | ++ + | + |
Focus of fibroid | + | + | + | ++ |
Discussion
Since TCs were reported for the first time, a number of studies worldwide have described these cells in different organs. Their primary identification is frequently sought. The c-kit receptor was the most attractive target in immunohistochemical observation. However, duplex immunoreactivity gave a reason to discuss a few populations of TCs in the human myometrium and the human small intestine [47–49]. In 2005, Ciontea et al. suggested that at least two subpopulations of TCs could exist in the human myometrium: c-kit-positive and c-kit-negative cells [48]. In addition, consistent with previous studies, Went et al. provided a list of c-kit-negative structures including the endometrium, myometrium and uterine cervix [50]. Moreover, Duquette et al. separately revealed vimentin (+)/c-kit (–) TCs in the human myometrium [47]. Later, in 2014, Yang et al. revealed that TCs were negative for c-kit in the rat oviduct [35]. First, Faussone-Pellegrini et al. concluded in 2011 that CD34-labeling “remains the best available choice for TC identification, possibly in combination with c-kit and vimentin labeling”, as c-kit-negative cells were described not only in the human uterus but also in the animal uterus [51]. Two years later, Vannucchi et al. proposed double immunofluorescent staining for CD34 and PDGFR-α as a specific marker for TCs in the gastrointestinal tract [52], which was successful in the heart and other organs [53]. Finally, in 2016, Faussone-Pellegrini et al. emphasized that “CD34 is the most reliable TC marker” [26]. Recently, some authors published the results of TC identification in the uterus based only on c-kit immunolabeling, which, in our opinion, is an omission. Moreover, some of them stressed that leiomyoma does not have any c-kit-positive cells, while an excess number of mast cells, common for the studied pathology, is strongly c-kit-positive. Therefore, we stressed the significance of using double immunofluorescent staining with anti-tryptase antibodies for separating mast cells from TCs. We found CD34- and PDGFR-α-positive cells in myometrial tissue from the foci of fibroids, the surrounding walls of the same uterus and healthy samples without UL. We emphasized that TCs exist in all samples of observed tissue.
Several studies have been devoted to explaining a possible role of new cells in diseases. In addition, all authors identified some quantitative and qualitative features common to TCs in pathological conditions: a reduction in numbers and/or damage of cells (up to absence) as well as shrinkage and shortening of Tps [26, 28, 54, 55]. Most likely, they are more sensitive to local ischemia than other stromal cell types, such as fibroblasts, myofibroblasts and mast cells. Manetti et al. observed the dynamics of TCs in tissues (the gastric wall – submucosa and muscle layers, the myocardium and the lung) from patients affected by systematic sclerosis and suggested that the reduction of TCs can lead to changing of the three-dimensional organization of the extracellular matrix and, as a result, the development of fibrosis [28]. In addition, the similar decline of TCs has been documented in myocardial infarction [30], aging of the human heart [56], liver fibrosis [37], ulcerative colitis [57], Crohn’s disease [29], gallstone disease [19], endometriosis [35], psoriasis [34], renal ischemia/reperfusion injury [40, 41] and different lung diseases [56]. Regardless of location, staging and character of pathology, the reduction of TCs is correlated with subsequent fibrosis, has a cellular origin and is common for many injuries and pathologies [58, 59]. We intend to provide further quantitative analysis of TC number in the foci of myoma and healthy myometrium, although we could hypothesize that the density of TCs declines in leiomyoma.
The interaction between TCs and the pathophysiological mechanisms common for the selected diseases is unclear. From one point of view, the amount of TCs declined under changes in the microenvironment associated with pathology. From the other viewpoint, the decrease of TCs could be a key point in the pathogenesis. These cells reflect damage, or they could be involved in the background of the diseases; the question remains open. We revealed the presence of TCs in uterine fibroids. Before, only comparisons of the density and the distribution in pregnant and nonpregnant uteruses in rats and humans had been provided. TCs constituted approximately 7% of the total cell number in nonpregnant myometrial cell culture and approximately 3% of the entire cell population in the myometrium of adult nonpregnant humans [60, 61]. The TC interstitial system is composed of cells that by either homocellular or heterocellular contact integrate overall information from the vascular, the nervous and the immune systems, the interstitium and stem cells. In the myometrium, TCs can influence the contractile activity of smooth muscle cells. Of note, they differed in telopodal width and podomic thickness with pregnancy states, which may be related to their function [44, 45]. Current studies showed that the podomers are thicker in nonpregnant myometrium than in pregnant myometrium (~82 vs. 75 nm), and the podoms were thicker in pregnant myometrium (~316 vs. 269 nm) [21, 43].
Richter et al. demonstrated that the numbers of TCs and Tps correlate negatively with the amount of mature fibrillar collagens and correlate positively with degraded collagen in the human heart [54]. We observed the same trend, which requires further investigation.
Growth factors have an influence on myometrial cellular transformation and turnover. For instance, PDGF modulates the rate of cell proliferation in myometrium and leiomyoma cells and likely plays a role in smooth muscle cell (SMC) hypertrophy as its expression is increased in the myometrium during gestation. It is upregulated by estrogen in uterine SMC and might interact with other growth factors such as TGF-β and EGF to enhance proliferation [5]. TCs are immunohistochemically positive for platelet-derived growth factor receptor α and β (PDGFR-α and-β) and VEGF. Of note, telocytes might be involved in the regulation of excessive cellular matrix production inside the foci of myoma [62].
Myometrial changes relate to three basic pathophysiological links: fibrosis, angiogenesis and the immune response. Growth factors involved in the pathogenesis of UL have receptors on TCs. Recent data showed that TCs are immunopositive to TGF-β, which is also involved in myocardial physiopathology. We suggest that the same could occur in myometrial contractility. One of the essential elements of leiomyomata is the vascular capsule. Neovascularization correlates with hypoxia and misbalance of vascular and coagulation factors. We observed a decrease of vascularization in the foci of leiomyoma, accompanied by increasing expression of VEGF receptor-1 (sFlt-1). Hypoxia could be a leading factor leading to this change. However, the common feature for both observed subjects was that in the foci of leiomyoma we saw a decline of telocytes and of vascularization too. Uterine telocytes are sensitive to angiogenic factors (PDGF and VEGF) and ischemia; they declined and even disappeared during fibrosis, observed in close vicinity to blood vessels. They might play a role in the angiogenic response, universal for the human body. In clinical practice it might clarify the pathogenesis of the oxidative response in the myometrium and help in selection of further appropriate therapy as a consequence. We intend to observe this correlation in our future scientific work.
In conclusion, our data demonstrate that TCs are present in human uterine fibroids and highlight their possible involvement in the pathogenesis of myometrial pathology in the context of angiogenesis. Further studies will allow clarification of the details of their putative roles in angiogenesis and the principles of their interaction in the myometrium. We hypothesize that in-depth observation of TCs in the human uterus brings additional value to reproductive medicine.