Introduction
Hepatocellular carcinoma (HCC) represents the fifth most common cancer and the most prevalent type of hepatobiliary carcinoma worldwide. The only potentially curative modality for HCC is surgical intervention, including resection, ablation, and transplantation, yet the recurrence rate remains high and the long-term survival rate is rather poor [1, 2].
Most currently available drugs for cancer therapy have a limited potential since they are extremely toxic, inefficient in treating cancer, or highly expensive and thus beyond the reach of most people. As a consequence of the limited progress attained by cancer chemotherapy over the past three decades, the interest of the researchers in the chemoprevention of cancer has been raised [3], especially using nutraceuticals originating from nutritional sources which are naturally multi-targeting, less expensive, safer and instantly available [4].
The derivatives of the polyphenol compounds are among the most valuable nutraceuticals that have antitumor effects. One of these derivatives is curcumin, which is obtained from the plant Curcuma longa, frequently called turmeric. It has gained enormous importance for its exceptional antioxidant, anti-inflammatory, anti-carcinogenic, and anti-angiogenic activities with no noticeable toxicity [5]. Accumulated experimental evidence implies that curcumin interferes with a variety of molecular targets and processes involved in cancer [6]. However, the therapeutic applications of curcumin in humans are constrained by its prominent metabolic instability as well as inadequate absorption and bioavailability [7].
Diethylnitrosamine (DEN) is a potent genotoxic nitrosamine that is known to induce damage of the nuclear enzymes involved in DNA repair/replication and is typically used in experimental models to cause liver cancer. It has been demonstrated that DEN is metabolized into its active ethyl radical metabolite that interacts with DNA causing its mutation and chromosomal aberrations which would eventually lead to the development of hepatocarcinogenesis [5]. Additionally, the active metabolites of DEN generated by cytochrome isoform 2E1 (CYP 2E1) increase the oxidative stress level, leading to cytotoxicity [8].
This study was designed to study the effect of curcumin, alone and in combination with nanoparticles (Nano Cur), as an attempt to increase its bioavailability and to investigate their therapeutic and preventive potential for DEN-induced HCC in an animal model.
Material and methods
Synthesis of nanoparticulate curcumin
Silver nanoparticles have been prepared by the chemical reduction method as reported by Pal et al. [9]. They were prepared by microwave irradiation of silver nitrate (AgNO3) solution in ethanolic medium using polyvinyl pyrrolidine (PVP) as a stabilizing agent. Ethanol was observed to act as a reducing agent in the presence of microwaves. The colorless solution instantaneously changed to a pale-yellow color, indicating the formation of silver nanoparticles. Characteristic optical properties of the silver nanoparticles were obtained using an Ocean Optics USB2000+VIS-NIR Fiber optics spectrophotometer. Shape and particle size were determined using a JEOL JEM-2100 high resolution transmission electron microscope (TEM) at an accelerating voltage of 200 kV. The TEM image showed the spherical silver nanoparticles of 12 ±2 nm diameter. The visible spectrum of silver nanoparticles showed a surface plasmon band at 411 nm. Then, the curcumin loading process was achieved via an ultra-sonication homogenizer using the stoichiometric ratio between silver nanoparticles and curcumin.
Animals and treatment
The animals used throughout this study were male CD1 albino mice (Mus musculus); their weights were ranged between 20 and 25 g. They were obtained from the Theodor Bilharz Research Institute (Imbaba, Giza, Egypt). Mice were acclimatized to laboratory conditions for 7 days prior to the commencement of the treatment. They received proper care and handling in compliance with the Animal Ethics Committee. The standard rodent food pellets contained maize, wheat bran, unhulled cottonseed, molasses, pulverized limestone, and salt. The percentages of crude proteins, fats, and fibers in the food pellets were 14%, 2%, and 15%, respectively.
Experimental design
Mice were randomly divided into eleven groups, with 6 animals in each group, for a study period of 15 weeks.
Group 1: normal (control) group, only received water and food (no treatment).
Group 2: injected intraperitoneally with N-nitrosodiethylamine (DEN) (Sigma-Aldrich co., Missouri, USA) once/week for 15 weeks at a dose of 15 mg/kg in the first 6 weeks and 25 mg/kg in the next 9 weeks.
Group 3: treated with free curcumin (LKT Laboratories, Minnesota, USA) orally by gavage at a dose of 50 mg/kg (high dose) twice/week for 15 weeks.
Group 4: treated with free curcumin orally at a dose of 10 mg/kg (low dose) twice/week for 15 weeks.
Group 5: injected with DEN as in group 2 plus high-dose free curcumin orally twice/week for 15 weeks.
Group 6: injected with DEN as in group 2 plus low-dose free curcumin orally twice/week for 15 weeks.
Group 7: treated with polyvinyl pyrrolidine (PVP) capped-silver nanoparticles orally as a control to nanoparticulate curcumin for 15 weeks twice/week.
Group 8: treated with nanoparticulate curcumin orally at a dose of 3.3 mg/kg (high dose) twice/week for 15 weeks.
Group 9: treated with nanoparticulate curcumin orally at a dose of 0.6 mg/kg (low dose) twice/week for 15 weeks.
Group 10: injected with DEN as in group 2 plus high-dose nanoparticulate curcumin orally twice/week for 15 weeks.
Group 11: injected with DEN as in group 2 plus low-dose nanoparticulate curcumin orally twice/week for 15 weeks.
Blood and tissue sampling
At the end of the experiment, the animals were sacrificed. The serum was separated from the mice blood, divided into aliquots and stored at –80°C until used for analysis. A portion of the liver was immediately fixed in 10% formalin for histopathological analysis and the rest of the liver was wrapped in aluminum foil and kept frozen at –80°C until used for analysis.
Biochemical analysis
Serum aspartate aminotransferase (AST), alanine aminotransferase (ALT), alkaline phosphatase (ALP), total protein (TP), and albumin (Alb) were chemically determined using the Beckman Coulter Synchron CX-5 Pro. System (Beckman Coulter, California, USA).
Measurement of serum levels of TNF-α, VEGF, and AFP
Serum tumor necrosis factor-alpha (TNF-α) levels were determined using platinum ELISA kit (eBioscience, San Diego, CA, USA), serum vascular endothelial growth factor (VEGF) levels were determined using a platinum ELISA kit (R&D Systems, Minneapolis, USA) and serum α-fetoprotein (AFP) levels were determined using a platinum ELISA kit (R&D Systems, Minneapolis, USA).
Estimation of liver antioxidant enzymes
Reduced glutathione (GSH) level and catalase (CAT), glutathione peroxidase (GPx), and superoxide dismutase (SOD) activities were measured colorimetrically using a biodiagnostic kit (Cairo, Egypt).
Estimation of liver oxidative stress markers
Liver nitric oxide (NO) and malondialdehyde (MDA) concentrations were measured colorimetrically using a biodiagnostic kit (Cairo, Egypt).
Histopathological procedure
Liver tissues previously fixed in 10% formalin were trimmed, washed, and dehydrated in ascending grades of alcohol. Tissue specimens were then cleared in xylene, embedded in paraffin, sectioned at 5 μm thickness, and stained with hematoxylin and eosin (H&E) to verify histological details.
Gene expression analysis by real-time PCR
Total RNA was extracted from homogenized liver samples using the QIAamp RNA Blood Mini Kit (Qiagen, USA). Concentration and purity of the extracted RNA were assessed by a NanoDrop 2000 spectrophotometer (Thermo Scientific, USA). Reverse transcripts were prepared from the extracted RNA using QuantiTect Reverse Transcription kit (Qiagen, USA). Gene expression was relatively quantified by real-time PCR using a QuantiTect SYBR Green PCR kit (Qiagen, USA). All the primers were ready-made provided by Invitrogen, USA. The relative quantification of the caspase-3, P53, and NF-κB was calculated against a reference gene, GAPDH. PCR reactions were performed with 2 μl of primer, 12.5 μl of 2x QuantiTect SYBR Green PCR Master Mix, 8.5 μl of RNase-free water, and 2 μl of cDNA. Cycling was initiated with 95°C for 15 min followed by 40 cycles of 94°C denaturation for 15 s, 55°C annealing for 30 s, and 72°C extension for 30 s, followed by a melt curve.
Statistical analysis
All the grouped data were evaluated with SPSS software (version 19). Hypothesis testing methods included one-way analysis of variance (ANOVA) followed by the least significant difference (LSD) test, which was used to identify differences between group means. P-values of < 0.05 were considered as the minimum level of significance. The data were expressed as mean ± SE with six animals in each group.
Results
Biochemical analysis
Effect on serum liver function tests
Intraperitoneal injection of mice with DEN caused a significant increase in ALT (p < 0.001), AST, and ALP activities (p < 0.01), and a significant decrease in the albumin level (p < 0.05), compared to the normal control group.
Oral treatment of DEN-injected mice with free and nanoparticulate curcumin led to a significant decline in ALT and ALP activities (p < 0.01) and a significant increase in albumin level (p < 0.01), compared to the DEN group. AST activity decreased significantly when DEN-injected mice were treated with high-dose free curcumin (p < 0.05) and both doses of nanoparticulate curcumin (p < 0.01), compared to the DEN group (Table I).
Table I
Results of liver function tests of the different studied groups
Groups | AST [IU/l] | ALT [IU/l] | ALP [IU/l] | TP [g/dl] | Alb [g/dl] |
---|---|---|---|---|---|
Normal control | 189.5 ±29.6 | 48.5 ±3.9 | 49.5 ±5.47 | 7.00 ±0.2 | 3.8 ±0.2 |
Nano control | 233.33 ±5.4 | 40.33 ±1.56 | 35.50 ±1.93 | 6.52 ±0.29 | 3.72 ±0.21 |
Free cur. HD | 242.0 ±13.39 | 42.17 ±2.74 | 40.33 ±1.33 | 6.30 ±0.10 | 3.62 ±0.06 |
Free cur. LD | 178.83 ±18.4 | 37.50 ±2.67 | 38.67 ±1.71 | 5.93 ±0.11 | 3.63 ±0.10 |
Nano cur. HD | 205.3 ±9.6 | 42.33 ±3.67 | 40.17 ±1.74 | 6.48 ±0.34 | 4.13 ±0.08 |
Nano cur. LD | 219.3 ±11.8 | 41.0 ±1.56 | 38.33 ±1.62 | 5.70 ±0.15 | 3.35 ±0.13 |
DEN | 322.50 ±21.0* | 114.0 ±17.04** | 66.67 ±6.94* | 6.80 ±0.2 | 3.25 ±0.19d |
DEN + Free cur. HD | 268.2 ±27.2b | 52.50 ±4.98a | 54.17 ±3.84a | 6.90 ±0.16 | 3.72 ±0.08e |
DEN + Free cur. LD | 305.50 ±38.70 | 53.7 ±3.9a | 49.0 ±2.7a | 6.53 ±0.28 | 3.85 ±0.2e |
DEN + Nano cur. HD | 248.5 ±14.0a | 43.17 ±2.36a | 33.33 ±2.47a | 6.55 ±0.24 | 3.63 ±0.14e |
DEN + Nano cur. LD | 251.2 ±24.5a | 38.67 ±3.04a | 42.50 ±5.21a | 6.73 ±0.14 | 3.78 ±0.09e |
Changes in levels of TNF-α, VEGF, and AFP
Injection with DEN induced a significant rise in TNF-α level (p < 0.01). Mice injected with DEN and treated with both doses of free curcumin and of nanoparticulate curcumin displayed a significant decrease in TNF-α level (p < 0.01), compared to the DEN group.
DEN inoculation was accompanied by a significant elevation in VEGF level (p < 0.01). Treatment of DEN-injected mice with free curcumin and nanoparticulate curcumin, both high and low doses, resulted in a significant decrease in VEGF levels (p < 0.01), compared to the DEN group.
AFP level was raised significantly (p < 0.01) when mice were infused with DEN. Both doses of free curcumin and of nanoparticulate curcumin induced a significant decline of AFP (p < 0.01) in comparison to the DEN group (Table II).
Table II
Results of TNF-α, VEGF, and AFP in the different studied groups
Groups | TNF-α [pg/ml] | VEGF [pg/ml] | AFP [ng/ml] |
---|---|---|---|
Normal control | 116.8 ±6.8 | 120.2 ±1.48 | 3.72 ±0.09 |
Nano control | 110.5 ±9.7 | 121.50 ±1.42 | 3.28 ±0.29 |
Free cur. HD | 103.3 ±9.2 | 126.42 ±1.53 | 4.00 ±0.27 |
Free cur. LD | 121.3 ±8.4 | 127.37 ±1.33 | 4.03 ±0.18 |
Nano cur. HD | 118.0 ±5.2 | 122.62 ±2.18 | 4.01 ±0.16 |
Nano cur. LD | 132.4 ±3.4 | 119.67 ±1.52 | 3.93 ±0.11 |
DEN | 484.9 ±94.3a | 531.27 ±7.76a | 55.72 ±0.89a |
DEN + Free cur. HD | 210.8 ±19.3** | 360.53 ±3.93** | 17.58 ±0.66** |
DEN + Free cur. LD | 238.1 ±39.9** | 401.38 ±6.58** | 19.57 ±0.59** |
DEN + Nano cur. HD | 142.8 ±11.8** | 191.07 ±2.84** | 9.12 ±0.26** |
DEN + Nano cur. LD | 179.3 ±15.7** | 256.90 ±3.37** | 14.58 ±0.59** |
Estimation of liver antioxidant enzymes
Injection with DEN decreased SOD activity significantly (p < 0.01). Treatment of DEN-injected mice with both doses of curcumin, either free or nanoparticulate, augmented the SOD activity significantly (p < 0.01), compared to the DEN-treated group.
DEN inoculation raised the GSH level significantly (p < 0.001) but both doses of free and of nanoparticulate curcumin reduced it significantly, in the injected mice (p < 0.001).
When DEN was injected, catalase activity fell significantly (p < 0.01). Treatment with free curcumin and nanoparticulate curcumin, either high doses or low doses, increased the catalase activity (p < 0.01), compared to the DEN-injected group.
Also, DEN caused a significant decrease in GPx activity (p < 0.01) and both doses of nanoparticulate curcumin elevated it significantly, in comparison to the DEN-injected group (p < 0.01) (Figure 1).
Figure 1
Results of liver antioxidant enzymes in the different studied groups. Values are expressed as mean ± standard errors represented by vertical bars
**p < 0.01 significance increase than DEN, ap < 0.01 significant decrease than normal, bp < 0.001 significant increase than normal, cp < 0.001 significant decrease than DEN, SOD – superoxide dismutase, GSH – glutathione reduced, GPx – glutathione peroxidase
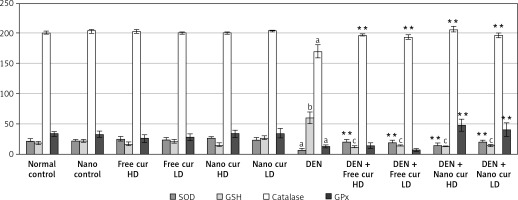
Estimation of liver oxidative stress markers
Malondialdehyde (MDA) level rose significantly when mice were injected with DEN (p < 0.01). Treatment with both doses of free curcumin (p < 0.05) or a low dose (p < 0.05) or a high dose (p < 0.01) of nanoparticulate curcumin in DEN-injected mice induced a significant decrease in MDA level.
A high dose of nanoparticulate curcumin caused a significant decrease in NO level, compared to the control group. Although injection of mice with DEN did not induce any difference in NO level, oral treatment with high-dose free curcumin in DEN-injected mice reduced the NO level significantly (p < 0.01) (Figure 2).
Figure 2
Results of liver oxidative stress markers in the different studied groups. Values are expressed as mean ± standard errors represented by vertical bars
ap < 0.01 significant increase than normal, dp < 0.01 significant decrease than normal, *p < 0.05 significant decrease than DEN, **p < 0.01 significant decrease than DEN, MDA – malondialdehyde, NO – nitric oxide.
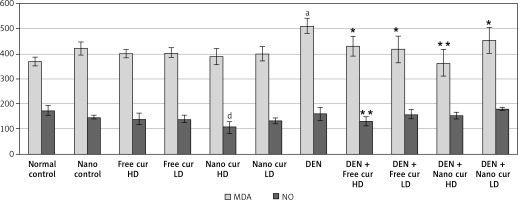
Histopathological examination of liver tissues stained with (H&E)
Hepatic tissues of normal control mice displayed hepatic lobules with intact lobular architecture and portal tracts are of normal shape and thickness (Figure 3 A).
Figure 3
A – Normal control showing normal hepatic architecture with radial arrangement of hepatocytes rays around the central vein (long arrows) that are separated by sinusoids (short arrows) (H&E 200×), B – mice treated with silver nanoparticles showing mildly disturbed architecture and congested veins with normal-sized hepatocytes (H&E 200×)
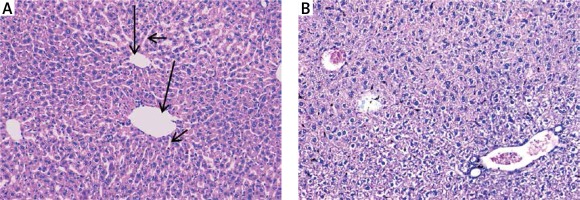
Silver nanoparticles-treated mice displayed mildly disturbed liver architecture, mildly congested veins, and normal appeared hepatocytes (Figure 3 B). Hepatic tissues of mice treated with either a high dose or a low dose of free curcumin showed a picture closely similar to the normal control mice (Figures 4 A and B). In mice treated with nanoparticulate curcumin, the lobular architecture was preserved with normally appearing hepatocytes and mildly congested veins (Figures 4 C and D).
Figure 4
Mice treated with: A – high-dose curcumin; B – low-dose curcumin; C – high-dose nanoparticulate curcumin; D – low-dose nanoparticulate curcumin show preserved lobular architecture and normally appeared hepatocytes (H&E 200×)
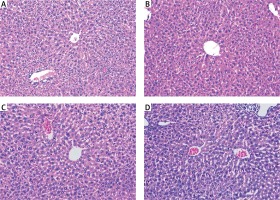
Loss of hepatic lobular architecture was observed in DEN-injected mice and liver tissue was composed of solid sheets of malignant cells with mild to moderate nuclear atypia, nuclear hyperchromasia, pleomorphism, and increased nucleo/cytoplasmic ratio. Some portal tracts showed proliferating bile ducts. There was focal necrosis with pericentral and periportal mononuclear inflammatory cell infiltration and intra-cellular cholestasis (Figure 5).
Figure 5
Hepatocellular carcinoma, grade 2: disturbed architecture; malignant hepatic cells with nuclear atypia and cholestasis (arrows) (H&E 200×)
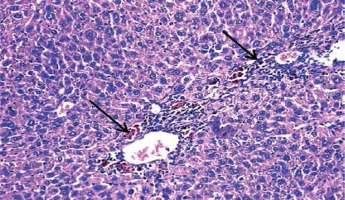
Mice injected with DEN and treated with a high dose of free curcumin were improved with restoration of normal lobular architecture and disappearance of inflammation and cholestasis (Figure 6 A). Hepatic tissues from mice injected with DEN and treated with a low dose of free curcumin showed regression of the malignant change. However, there was disturbed architecture with resistant few foci of inflammation and cholestasis (Figure 6 B).
Figure 6
Mice injected with DEN and treated with: A – high-dose curcumin show regression of malignant change with almost restoration of lobular architecture; B – low-dose curcumin show regression of malignant change with disturbed architecture and resistant few foci of cholestasis (arrows); C – high-dose nanocurcumin; D – low-dose nanocurcumin show restoration of lobular architecture and marked reduction in the extent of inflammation and cholestasis (H&E 200×)
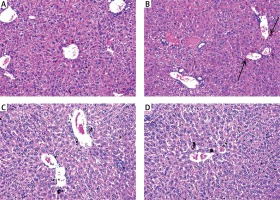
Mice injected with DEN and then treated with a high dose of nanoparticulate curcumin showed restoration of the normal lobular architecture, an improvement in the cytological features, and disappearance of cholestasis and inflammation (Figure 6 C). Hepatic tissues of mice injected with DEN and treated with a low dose of nanoparticulate curcumin showed restoration of normal lobular hepatic architecture and a marked reduction in the extent of cholestasis and inflammation (Figure 6 D).
NF-κB, caspase-3, and P53 gene expression
Silver nanoparticle treatment upregulated NF-κB expression (p < 0.05), compared to the normal control group. When mice were injected with DEN, a significant increase in NF-κB (p < 0.01) was observed. Treatment of DEN-injected mice with both doses of free curcumin and of nanoparticulate curcumin downregulated NF-κB significantly (p < 0.01), compared to the DEN group.
Injection with DEN had no significant effect on caspase-3 mRNA level (p > 0.05), compared to the normal control group. Treatment with high-dose nanoparticulate curcumin in DEN-injected mice resulted in a significant rise in caspase-3 (p < 0.01), in comparison to the DEN group.
On injection of mice with DEN, tumor suppressor P53 level was upregulated significantly (p < 0.01). Free curcumin and nanoparticulate curcumin, either high doses or low doses, led to a significant decrease in P53 expression in DEN-injected mice (p < 0.01) (Figure 7).
Figure 7
Results of gene expression analysis of NF-κB, caspase-3, and P53 in the different studied groups. Values are expressed as mean ± standard errors represented by vertical bars
ap < 0.05 significant increase than normal, bp < 0.01 significant increase than normal, cp < 0.01 significant decrease than DEN, dp < 0.01 significant increase than DEN, NF-κB – nuclear factor κB, P53 – tumor suppressor P53.
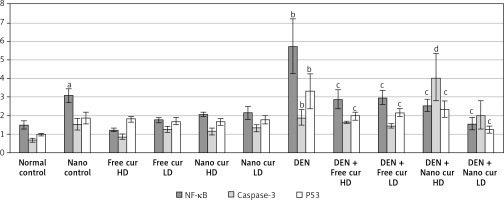
Discussion
Hepatocellular carcinoma (HCC), the most prevalent form of primary liver malignancy, is the third leading cause of cancer mortality worldwide, with more than 500,000 people affected annually [2, 10]. Several treatment modalities exist for HCC. However, the recurrence rates are as high as 50%. Moreover, HCC is widely considered as a chemotherapy-resistant disease. In view of these reasons, chemoprevention has been suggested as the paramount approach in lowering the occurrence and mortality associated with HCC [11].
It has been revealed that curcumin has cancer-inhibitory effects on many types of malignancies affecting the reproductive, digestive, urinary, pulmonary, and nervous systems. Also, it has a protective effect against the development of conditions linked to inflammation such as psoriasis and Alzheimer’s disease [12]. However, the therapeutic applications of curcumin are restricted by its metabolic instability as well as poor absorption and bioavailability [7]. Therefore, nanoparticles were used in this study to overcome these difficulties as they are known to accumulate mostly at tumor sites because of the poor lymphatic drainage of macromolecules in solid tumors and the retention power of cancer cells [13].
To clarify the role of curcumin and nanoparticulate curcumin in the prevention of HCC, we established a model of diethylnitrosamine (DEN)-induced HCC in male Albino mice. Our data revealed that DEN administration provoked a significant increase in the serum activities of AST, ALT, and ALP enzymes indicating liver cell damage. On the other hand, free and nanoparticulate curcumin administration decreased the activities of AST, ALT, and ALP enzymes, compared to the HCC group. This reduction may be attributed to the diminution of hepatocellular damage [14], suggesting that curcumin might have protective potential against DEN-induced liver damage.
DEN infusion significantly decreased serum albumin levels which implies that the synthetic liver functions fell whereas curcumin and nanoparticulate curcumin treatment increased the serum albumin levels.
Compared to the normal control group, DEN-treated mice showed a significant fall in the activities of glutathione peroxidase (GPx), superoxide dismutase (SOD), and catalase (CAT). Also, a significant increase in malondialdehyde (MDA) and reduced glutathione (GSH) levels were observed, indicating enhanced generation of free radicals as a result of DEN injection, which disrupts the antioxidant defense systems and increases the reactive oxygen species (ROS) and membrane lipid peroxidation [15]. In contrast, curcumin administration resulted in a drop of the oxidative stress induced by DEN. Levels of MDA and GSH were significantly declined in curcumin-administered groups while there was a significant elevation in GPx, SOD, and CAT activities, compared to the HCC group.
Curcumin is a potent scavenger of free radicals such as superoxide anions, nitrogen dioxides, and hydroxyl radicals [16]. So, it may lower the heterocyclic aromatic amine toxicity and can inhibit the hepatocarcinogenic effect of nitrosamines. In line with our results, Notarbartolo et al. [17] concluded that curcumin causes cell growth inhibition and apoptotic effects in hepatic cancer cell line.
Our findings compare favorably with Khan et al. [18], who reported that alpha-fetoprotein (AFP) serum level was elevated in DEN-treated mice. Curcumin treatment decreased its level considerably.
Serum levels of VEGF were elevated in DEN-injected mice. This agreed with Mazzocca et al. [19], who stated that VEGF is a strong angiogenic factor and is mainly responsible for the neovascularization in HCC. Free and nanoparticulate curcumin administration decreased serum levels of VEGF, thereby inhibiting the formation of new blood vessels and tumor growth. This was very similar to the findings of Yoysungnoen et al. [20], who observed that curcumin administration decreased the levels of the angiogenic biomarker VEGF in HCC and reduced the density of tumor neovascularization. Similarly, Pan et al. [21] detected that curcumin significantly restrained HCC development in vitro and in vivo by targeting VEGF expression.
Curcumin decreased TNF-α serum levels and NF-κB expression. This was in agreement with previous reports which showed that the therapeutic effect of curcumin may be attributed to its down-regulation of NF-κB through the suppression of IκBα kinase (IKK) activation that halts cell growth and provokes apoptosis. Furthermore, curcumin treatment diminished TNF-α level, which led to the inhibition of NF-κB and consecutively, prevention of cell proliferation [22].
Our results established that the administration of high-dose nanoparticulate curcumin significantly upregulated caspase-3 expression, compared to the DEN group, which agreed with Anto et al. [23], who stated that curcumin induced apoptosis through the mitochondrial pathway involving cytochrome c release and caspase-3 activation in HL-60 cells of human AML.
In DEN-injected mice, the tumor suppressor P53 was up-regulated. This may be due to the formation of different types of DNA adducts, which have different effects on distortion of the DNA helix, DNA replication, and on the induction of gene mutations which induces expression of the P53 gene.
Curcumin administration decreased P53 expression in DEN-infused mice, which agreed with Bush et al. [24], who illustrated that curcumin induced apoptosis in human melanoma cells through the Fas/caspase-8 pathway independent of p53 and they found that the level of expression of P53 was not influenced by curcumin. In contrast, other studies showed that curcumin promoted apoptosis through p53 upregulation in glioma, prostate, and breast cancer cell lines [25].
Changes in the biochemical parameters and gene expression were confirmed by the histopathological examination. The liver tissues of DEN-injected mice showed a malignant picture, consistent with previous studies [26], with loss of hepatic lobular architecture while treatment with either high-dose curcumin or both doses of nanoparticulate curcumin revealed an improvement with restoration of normal lobular architecture and disappearance of inflammation and cholestasis.
In conclusion, this study proves that curcumin has potent anti-oxidant, anti-inflammatory, anti-carcinogenic, and anti-angiogenic activities and either the high dose of free curcumin or the tested doses of nanoparticulate curcumin are effective in preventing DEN-induced liver carcinoma. Moreover, it is obvious that nanoparticles improve curcumin absorption and bioavailability as they were effective in preventing liver carcinoma despite their extremely low doses, in comparison to free curcumin. Consequently, with the development of these drug delivery nanoparticles, the drawbacks caused by the low bioavailability of curcumin can be overcome and there will be no need to raise the curcumin dose to become effective.