Introduction
Head and neck squamous cell carcinoma (HNSCC) is the most common type of malignant cancer in the head and neck region, with a globally reported annual incidence of approximately 550 000 patients [1]. While advances have been made in the radiotherapeutic, chemotherapeutic, and surgical treatments of HNSCC, including proton beam therapy, heavy ion therapy, molecular targeting therapy, and endoscopic laryngo-pharyngeal surgery, no marked improvements in the survival rates have been achieved [2, 3]. Accordingly, a new and effective method of treatment needs to be developed to increase the survival rate of patients with HNSCC. At our facility, we treat HNSCC using chemoradiotherapy and surgical treatment. However, recurrence and metastasis are frequently observed. One possible cause of this is that a small population of cancer stem cells (CSCs) found within tumors that acquire self-renewal and differentiation capacity develop resistance to therapeutics and act as a source of cancer cells that make up the tumor. Pharmaceutical agent and radiotherapy resistance make CSCs potential targets of cancer treatment. Recently, Sanne et al. reported CD98 as a stem cell marker of HNSCC [4].
CD98 is a cell surface antigen that forms a heterodimer of heavy and light chains connected by disulfide bonds. Heavy chains are bound to β integrin chains and play an important role in integrin signal amplification, and light chains function as amino acid transporters. One of the light chains – LAT1 – is a twelve transmembrane amino acid transporter. CD98 heavy and light chains transport several essential amino acids, i.e., leucine, isoleucine, valine, phenylalanine, tyrosine, tryptophan, methionine, and histidine. Among these, leucine controls translation, transcription, and cell growth via mTOR signaling. Heavy and light chain functioning allows CD98 to control cell proliferation, survival, migration, and adhesion [5] and is essential for B and T cell proliferation in the blood cell system [6, 7]. Further, CD98 overexpression correlates with metastasis and poor prognosis in several types of solid cancers [8, 9].
In the present study, we investigated whether CD98 heavy chains in HNSCC can be used as a marker for CSCs and whether they contribute to radiotherapy resistance and malignancy.
Material and methods
Cell culture
We used HO-1-u-1 (mouth floor); Sa3 (gingiva); and HSC2, HSC3, and HSC4 (tongue) cell lines. We purchased each cell line from the Riken BioResource Research Center. We maintained cell monolayers in RPMI 1640 medium (Nissui Pharmaceutical, Tokyo, Japan) supplemented with 10% fetal bovine serum (FBS, Clontech Laboratories, Mountain View, CA), 2 mM L-glutamine, 2.4 g/l NaHCO3, 100 U/ml penicillin, and 100 μg/ml streptomycin. The medium was changed every other day. For several experiments, serum-free RPMI 1640 medium supplemented with 2 mM L-glutamine, 2.4 g/l NaHCO3, 100 U/ml penicillin, 100 μg/ml streptomycin, 5.2 ng/ml sodium selenite, 0.025 mg/ml insulin, 20 ng/ml epidermal growth factor (EGF), and 10 ng/ml fibroblast growth factor (FGF) was used.
Establishment of radiation-resistant cell lines
All five cell lines were seeded in 25 cm2 flasks, and radiation (M-80WE, Softex, Japan) was continuously applied at 2 Gy on consecutive days until a dose of 60 Gy was reached. Surviving cells were passaged to establish radiation-resistant cell lines.
Flow cytometry
FITC-conjugated anti-human CD98hc mAb (eBioscience, Vienna, Austria) was added at a ratio of 1 : 11 to each single cell suspension containing 1 × 106 cells and incubated for 15 min at 4°C. After washing with phosphate buffered saline (PBS), propidium iodide (PI) was added to remove dead cells, and flow cytometry was performed using a BD FACS Aria III instrument (Becton, Dickinson and Company Japan, Tokyo, Japan).
Sphere formation assay
To avoid adhesion and subsequent maturation to non-CSCs, cells were cultured in a serum-free semisolid medium. In total, 1 × 103 cells for each of CD98hc-positive and CD98hc-negative fractions were seeded in 80 μl of serum-free RPMI 1640 medium supplemented as described above, containing 0.33% agar on 100 μl of a solidified serum-free RPMI 1640 basal layer containing 0.5% agar, in the wells of a 96-well plate. Fifty microliters of serum-free RPMI 1640 medium containing EGF and FGF was further poured on top of each well as a reservoir of fresh medium and growth factor, and this top liquid layer was changed every 3 days. Cells were incubated for 20 days in the same atmosphere as that for the monolayer culture. At the end of the 20-day incubation period, the generated tumor spheroids were counted.
Scratch wound healing assay
Cells were seeded in a 60-mm culture dish. After they reached confluence, an artificial wound was created by scratching the cell monolayer with 200-μl-pipette tips. Cells were cultured in a serum-free RPMI 1640 medium and incubated for 48 h. Next, cell migration was quantified using Image J.
Assessment of plating efficiency
FITC-conjugated anti-human CD98hc mAb (eBioscience) was added to each single cell suspension at a ratio of 1 : 11, and cells were incubated for 15 min at 4°C. Next, cells were washed with PBS, and PI was added to remove dead cells. CD98hc-positive and CD98hc-negative cells were sorted on a FACS Aria III instrument, and plating efficiency was determined. Briefly, 1 × 103 cells each of CD98hc-positive and CD98hc-negative cells were seeded onto 60-mm culture dishes containing RPMI 1640 medium supplemented as detailed in previous sections. After 7 days of incubation, cells were stained with hematoxylin. For both CD98hc-positive and CD98hc-negative cells, the number of cells was counted in five different 1-cm2 areas of each culture dish in triplicate. This value was then used to estimate the total number of cells in 60-mm culture dishes.
Cell invasion assay
FITC-conjugated anti-human CD98hc mAb (eBioscience) was added to each single cell suspension at a ratio of 1 : 11, and cells were incubated for 15 min at 4°C. Next, cells were washed with PBS, and PI was added to remove dead cells. CD98hc-positive and CD98hc-negative cells were sorted using a FACS Aria III instrument. Next, cell invasiveness was quantified using a Boyden chamber assay as described previously [10]. Briefly, 1 × 104 single-cell suspensions of both CD98hc-positive and CD98hc-negative cells were inoculated on Matrigel-coated membranes in a Boyden chamber. After 48 h, cells that moved from the upper chamber, passed through the Matrigel, and attached to the underside of the Matrigel-coated Boyden membrane were stained with hematoxylin and counted.
Establishment of tumor xenografts in immunodeficient mice
Male nude mice (BALB/c-nu) were purchased from Charles River Laboratories Japan (Yokohama, Japan) and maintained under specific pathogen-free conditions. The protocol of animal experiments was approved by the Animal Research Committee of Akita University and was in accordance with the university guidelines for the Regulation of Animal Experimentation. Nude mice were subcutaneously injected in the left and right flanks with 1 × 105 cells in 200 μl each of CD98hc-positive and CD98hc-negative cells, respectively. Tumorigenicity of cells was monitored for 1 month.
Immunohistochemistry
Formalin fixed paraffin-embedded sections on slide glass were deparaffinized, then immersed in 3% hydrogen peroxide/methanol at room temperature for 15 min. The slides were incubated with anti-CD98 mAb clone F-2 (Santa Cruz Biotechnology, Dallas, TX, USA) in a humidified chamber at 4°C overnight. Specific signals were visualized by employing the HRP-labeled polymer method as follows: the slides were reacted with EnVision+ system-HRP for mouse (Agilent, Santa Clara, CA, USA) at room temperature for 30 min and finally 3,3′-diaminobenzidine was oxidized for signal detection.
Results
Expression of CD98hc is drastically enhanced after acquisition of radiation resistance
Flow cytometry was performed to compare CD98hc expression between radiation-resistant and non-resistant cell lines. All radiation-resistant cells were > 80–90% positive for CD98hc expression (Figure 1). Since the expression level of CD98hc significantly increased after acquisition of radiation resistance in all the cell lines examined, the CD98hc-positive fraction in a population of cancer cells may represent radiation resistance and may have been predominantly selected through 60-Gy-irradiation. It is known that CSCs are characteristically prone to radiation resistance [11]. Furthermore, it has recently been proposed that CD98hc could be a CSC marker. We thus relevantly assume that CD98hc can be used as a marker of radiation-resistant CSCs. Elevated CD98hc expression is expected to result in elevated amino acid transporter expression, which would be extremely advantageous for cancer survival.
Figure 1
Radiation-resistant cell lines exhibiting elevated CD98hc expression. Flow cytometry was performed on all five parental and radiation-resistant cell lines to analyze CD98hc-positive and CD98hc-negative cell populations. A comparison of parental and radiation-resistant cells of each cell line showed that the CD98hc-positive population was significantly larger in the resistant cells than in the parental cells (p < 0.01)
SSC – side scattered light, RT – radiation-treated.
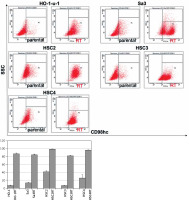
Radiation-resistant cell lines form many spheres
CSCs as well as normal tissue stem cells repeatedly undergo anchorage-independent self-renewal in a serum-free medium on a low- or non-attachment dish, enabling them to form multiple spheres [12]. We cultured the five cell lines in a semi-solid serum-free medium on a 96-well plate to investigate their ability to form stem cell spheres. Even in a serum-free semi-solid medium, radiation-resistant cell lines were able to form more spheres than non-resistant cell lines (Figure 2). A possible reason for this could be that irradiation enriched the CSC population.
Figure 2
Enhanced sphere formation in radiation-resistant cell lines. Radiation-resistant cell lines were able to form a higher number of spheres than non-resistant parental cells in serum-free medium (p < 0.05), indicating that irradiation elevated the proportion of CSCs in each cell line
RT – radiation-treated.
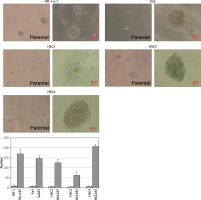
Radiation-resistant cell lines exhibit increased migration ability
All cell lines were seeded in a 60-mm dish, and an artificial wound was created by scratching the monolayer surface with 200-μl-pipette tips once the cells reached confluence. After culturing for 24 h in a serum-free RPMI 1640 medium, cell lines were assessed for their migration ability. Cell migration ability of the radiation-resistant cell lines was greatly enhanced (Figure 3). Since the phenotype of epithelial-mesenchymal transition including high motility has been considered to be one of CSCs’ hallmarks [13], expression of CD98hc may be involved in both stemness of CSCs and radiation resistance.
Figure 3
Enhanced migration ability in radiation-resistant cell lines. Wound healing assay was performed in serum-free medium to assess the effect of radiation resistance on migration ability. Significantly enhanced migration ability was observed in each cell line compared with that in non-resistant parental cells (p < 0.05)
RT – radiation-treated.
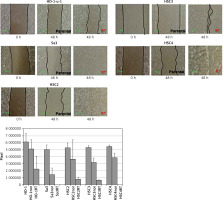
CD98hc-positive cells exhibit enhanced sphere formation ability
Even before irradiation, CD98hc-positive cells are contained as an absolute minority in all the cell lines examined including HO-1-u-1, Sa3, HSC2, HSC3, and HSC4 cells (Figure 1). Each of these different cell lines was sorted by FACS without irradiation to obtain CD98hc-positive and -negative fractions and two fractions from each cell line were compared in terms of sphere formation ability and plating efficiency in a serum-free semi-solid medium. As shown in Figures 4 and 5, sphere formation and plating efficiency were highly enhanced in CD98hc-positive cells compared with CD98hc-negative cells in all the cell lines. These results indicate that CD98hc-positive cells have characteristics of CSCs regardless of irradiation.
Figure 4
CD98hc-positive cells exhibiting enhanced tumor spheroid formation. CD98hc-positive and -negative cells were cultured in a serum-free semisolid medium on 96-well plates at a density of 1 × 103 cells/well. CD98hc-positive cells exhibited tumor spheroid formation ability, whereas CD98hc-negative cells did not (p < 0.01)
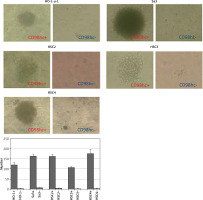
Figure 5
CD98hc-positive cells exhibiting enhanced invasiveness. CD98hc-positive and -negative cells were seeded onto Matrigel that had been poured into cell culture inserts in advance. Cells that invaded the Matrigel and attached to the underside of the membrane were counted after 48 h. Invasiveness of CD98hc-positive cells was significantly higher than that of CD98hc-negative cells (p < 0.01)
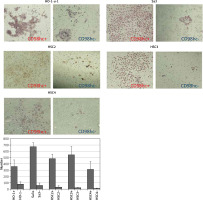
CD98hc-positive cells exhibit enhanced invasion ability
Using a Boyden chamber, non-irradiated CD98hc-positive and -negative fractions from each of the same cell lines as Figures 4 and 5 were compared in terms of invasiveness of Matrigel base membrane. As shown in Figure 6, CD98hc-positive cells were able to pass through the Matrigel more efficiently than CD98hc-negative cells, thereby confirming the enhanced invasiveness of CD98hc-positive cells.
Figure 6
CD98hc-positive cells exhibiting higher plating efficiency than CD98hc-negative cells. Cells from all cell lines were seeded at a density of 1 × 103 cells/60-mm dish and incubated for 7 days. Then, they were fixed with formalin and stained with hematoxylin. A higher plating efficiency was observed for CD98hc-positive cells than for CD98hc-negative cells (p < 0.05)
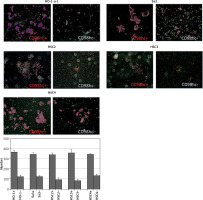
CD98hc-positive cells exhibit high tumorigenicity in vivo
Each of the Sa3 and HSC3 cell lines was sorted by FACS to obtain CD98hc-positive and -negative fractions. Each fraction was subcutaneously injected into athymic nude mice. CSCs are sometimes referred as “tumor initiating cells” and their experimental ultimate definition is a population of cancer cells that is capable of developing a tumor when xenografted in animals such as immunodeficient mice. In our experiments, CD98hc-positive cells were able to form a tumor in all the mice injected, whereas CD98hc-negative cells could not do so in any mice (Figure 7 A). Thus, it was confirmed that CSCs reside among CD98hc-positive cells but not among CD98hc-negative cells. We also injected the unsorted bulk of these two cell lines into nude mice and confirmed tumor formation in all the mice examined (data not shown). Furthermore, we immunohistochemically examined the expression level of CD98hc in the developed tumors from the CD98hc-positive fraction and unsorted bulk of Sa3 cells. As shown in Figure 7 B, while only a few cells give faint signals of CD98hc in the bulk-derived tumor, intensive signals are obvious in many tumor cells derived from the CD98hc-positive fraction.
Figure 7
CD98hc-positive cells but not CD98hc-negative cells formed tumors in vivo. In total, 1 × 105 cells each of CD98hc-positive and -negative cells from Sa3 and HSC3 were subcutaneously injected into the right and left flanks of male nude mice, respectively. While tumor formation was observed in the region where CD98hc-positive cells were injected, no palpable tumors were detected in the region where CD98hc-negative cells were injected (A). As revealed by immunohistochemistry, while high CD98hc expression was detected in the tumor developed by the CD98hc-positive fraction of Sa3 cells, the tumor developed by unsorted Sa3 cells expressed a much smaller amount of CD98hc in much fewer cells compared with the CD98hc-positive fraction-derived tumor (B)
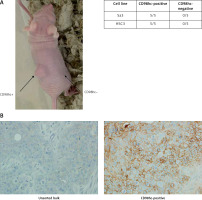
Discussion
Over the past several decades, marked advances have been made in surgical, radiotherapeutic, and chemotherapeutic treatment of HNSCC. Despite these advances, there have been no major improvements in the survival rates, which may be attributed to the presence of CSCs. Prince et al. [14] investigated the presence of stem cells in HNSCC and reported that tumors that formed as a result of the transplantation of clinical specimens from nine human patients into SCID mice contained CD44-positive cells that exhibited strong self-renewal ability and tumorigenicity. Wang et al. used SP cells in a similar investigation [15], and Chiou et al. reported that sphere-forming cells isolated from oral cancer cell lines expressed Oct-4, Nanog, CD117, Nestin, CD133, and ABCG2, with strong differentiation and invasion abilities [16]. However, markers of CSCs remain to be identified.
In recent years, CD98 has attracted attention. It has actually been established that CD98 is a poor prognostic factor in pancreatic carcinoma and non-small cell lung carcinoma, as proven by multivariate analyses based on clinical specimens from patients [17, 18]. As for pulmonary, colorectal, breast, and head-and-neck carcinomas and soft tissue sarcoma, it has been reported that CD98 is overexpressed in metastatic foci compared with their corresponding primary tumors [19]. In the present study, we investigated CD98hc expression in five HNSCC cell lines after applying irradiation at a total dose of 60 Gy. Interestingly, a marked increase in CD98hc expression was observed in all five cell lines after their acquisition of radiation resistance. Furthermore, since the CD98hc-positive but not -negative fraction could develop tumor in mice (Figure 7 A), it is certain that CSCs belong exclusively to the CD98hc-positive fraction. We thus successfully proved that the increased expression of CD98hc was a prerequisite for there being CSCs in the five cell lines of HNSCC. Taken together, although not all CD98hc-positive cells are CSCs or radiation-resistant, CD98hc is practically considered to be a biomarker for radiation-resistant CSCs.
Our finding that cells with CD98hc expression and radiation resistance showed enhanced motility even when cultured in serum-free medium was consistent with our empirical findings that cancer cells often spread much further than expected in patients suffering from recurrence after curative radiotherapy. In addition, we investigated whether CD98hc-positive cells exhibited properties of CSCs after sorting cells into CD98hc-positive and CD98hc-negative populations. The totality of our results, including the enhanced sphere formation ability, invasion ability, colony formation ability, and in vivo tumorigenicity of CD98hc-positive cell populations, indicates that the behaviors of CD98hc-positive cells are consistent with different properties of CSCs even if each behavior is not necessarily specific for CSCs. To the best of our knowledge, the present study is the first to compare between CD98hc-positive and CD98hc-negative cells in five different HNSCC cell lines and obtain consistent results.
CD98 is a heterodimer comprising heavy and light chains. The heavy chain binds to β-integrin and plays an important role in integrin signal amplification, cell proliferation, survival, migration, and adhesion [20–22], and the light chain is an amino acid transporter LAT1 that maintains rapid cell proliferation and enhanced intracellular metabolism in cancer cells. In this context, CD98hc functions as a co-factor stabilizing LAT1 and xCT [23]. Currently, LAT1-targeted cancer therapy is under investigation [24]. While CD98hc supports the function of LAT1, it has not been reported that LAT1 is involved in regulation of radiation sensitivity or cancer stemness, suggesting that radiation resistance and cancer stemness might be CD98hc’s own function independent of LAT1. Stabilization of xCT requires the binding of both CD98hc and CD44v9. Moreover, elevated xCT expression in tumors is strongly associated with CSCs in several tumors [25]. In a previous study, we demonstrated that irradiating HO-1-u-1, Sa3, HSC2, HSC3, and HSC4 cell lines resulted in CD44v9 overexpression [26]. In addition to CD98hc, the involvement of CD98lc and CD44v9 in radiation resistance will need to be clarified in future studies.
Our findings suggest that CD98hc-positive cells exhibit properties of CSCs and that CD98hc expression can serve as a potential marker for radiation resistance. The investigation of mechanisms underlying CD98hc expression and how it confers radiation resistance, as well as mechanisms underlying CD98hc, LAT1, xCT, and CD44v9 interactions, may lead to improved survival rates in patients with HNSCC.
In conclusion, in the present study, we demonstrated that irradiation of HNSCC cell lines resulted in CD98hc overexpression. CD98hc-positive cells were more likely to form tumor spheroids in a serum-free medium and exhibited higher in vivo tumorigenicity than CD98hc-negative cells. In the light of these findings, it has been revealed that CD98hc is profoundly related to characteristics of CSCs and radiation resistance. Therefore, it is proposed that CD98hc could be a useful marker to monitor the effects of CSC-targeting therapies, which are today being developed all over the world.