Introduction
Mammalian spermatozoa progressively acquire motility and fertilizing abilities during their transport, protection and storage in the epididymis. Therefore, secretion and reabsorption carried out by the epithelium lining of the epididymis are crucial in creating an optimal fluid microenvironment for post-testicular sperm maturation [1–4]. During transit along the epididymis, spermatozoa are potentially exposed to the harmful effects of reactive oxygen species (ROS) [5, 6]. High concentrations of ROS in the epididymis are due to the high metabolic activity of epididymal epithelial cells and a rich network of blood vessels surrounding the ductus epididymis. Reactive oxygen species are locally generated as a by-product of prostaglandin [7] and steroid hormone [8] synthesis. Another direct source of ROS are spermatozoa [9]. Although small and controlled amounts of free radicals appear to be essential for normal physiological functioning of male germ cells (proper spermatozoa DNA condensation, sperm hyperactivation, motility, capacitation and acrosome reaction) [10, 11], the exposure of spermatozoa to a high level of ROS critically impairs their function [5, 6, 12–15]. Due to the ambiguous nature of ROS, maintaining their risk-benefit balance is crucial for correct sperm physiology. The concentrations of antioxidants, well known to play a crucial role in minimizing oxidative damage in spermatozoa [16, 17], are limited due to the low amount of cytoplasm [18] and so are inadequate for full spermatozoa self-protection [5, 15, 16, 19]. Therefore, increased attention has been paid to the epididymis as a supportive organ protecting male reproductive cells against oxidative stress [16], and a rich source of antioxidant enzymes, e.g. catalase (CAT), superoxide dismutase (SOD), and enzymes associated with glutathione peroxidation and reduction, such as glutathione peroxidase (GPX) [16, 20–28] and glutathione reductase (GR) [29].
Almost half of the expression of proteins synthesized in the epididymis is regulated by androgens [30], the transcriptional factors for genes encoding antioxidant enzymes such as GPX [24, 31–35], SOD and CAT [36]. In our previous study we showed that treatment with finasteride (an inhibitor of 5α-reductase type 2) in adult male rats influenced the patterns of E-SOD, GPX5, and Cu/ZnSOD expression; that change was region-dependent and the expression was up- or down-regulated compared to control animals [37]. Recently, we also found that finasteride treatment of paternal rats resulted in altered serum and intratesticular levels of testosterone (T) and dihydrotestosterone (DHT) in their male offspring [38, 39]. Consequently, it can be speculated that finasteride, a drug that modulates the activity of the key enzyme involved in the metabolism of T, can act in a similar fashion to endocrine disruptors (EDs), and can negatively influence epididymal protection of spermatozoa against oxidative stress. As demonstrated by Parlaktas et al. [40], the alterations in antioxidant ROS scavenging capacities of the variants of antioxidant enzymes could be related to prostate cancer development.
In the light of the above described data, the aim of the experiment was to analyze the influence of androgen imbalances on the antioxidant protective functions of the epididymis. In this work, special attention was paid to cytoplasmic Cu/Zn-superoxide dismutase (SOD1), secretory epididymal-specific glutathione peroxidase (GPX5), CAT and GR, because together they constitute the “enzymatic protective system” used by the cells to resist reactive oxygen species. We studied the expression of CAT, SOD1, GPX5 and GR mRNA, and the immunolocalization of the proteins of these enzymes within the epididymis during neonatal/postnatal maturation in immature 7-, 14-, 21-, 28- and mature 90-day-old rat offspring (F1:Fin) born from females fertilized by finasteride-treated males. Additionally, the levels of antioxidant enzyme transcripts were correlated with blood serum concentrations of T and DHT with respect to animal age.
Material and methods
Animals
The study was conducted on albino Wistar rats in accordance with Polish law and with the approval of the Local Ethics Committee for Scientific Experiments on Animals in Szczecin, Poland (Resolution no. 23/2010).
Parent generation F0 produced male generation F1. Paternal rats in the F0:Fin group (n = 5) were treated with finasteride (Proscar, MSD, Cramlington, UK) in daily doses of 5 mg/kg/bw, according to our previous studies [41–43] and experiments by other researchers [44, 45]. The period of finasteride treatment before mating lasted 56 days (this period changed morphology and function of the testis [41–43]). The filial generation was collected over a period of 5 months to avoid ageing of the parent generation. Male rats (F0:Fin) received finasteride up to the end of the experiment (for 4–5 months). Once a week, the animals were weighed and the finasteride doses adjusted.
Generation of filial animals
The control group of offspring (F1:Control, n = 25) comprised male rats born from females fertilized by untreated control male rats. The experimental group of offspring (F1:Fin, n = 25) comprised male rats born from females fertilized by finasteride-treated male rats. The objective of the experiment was to sample the epididymis from both the treated and untreated offspring (F1) at 7, 14, 21, 28, and 90 postnatal days (PND) of life. Detailed information on the design of the animal treatment, mating, and collection of newborn offspring has been presented in our previous report [38]. After thiopental anesthesia (120 mg/kg bw, i.p., Biochemie GmbH, Vienna, Austria), the epididymides were weighed and the right epididymides (5 animals per age group in the F1 generation) used for immunohistochemical (IHC) reactions, and the left epididymides frozen and used for qRT-PCR analysis.
Quantitative real-time reverse transcription PCR (qRT-PCR) analysis
Quantitative analysis of mRNA expression of Cat, Sod1, Gpx5 and Gsr was performed in a two-step reverse transcription PCR. Tissues were suspended in 600 µl of RLT buffer and homogenized for 4 min on ice. Next, the sample was digested with proteinase K for 15 min at 55°C and isolated on spin columns according to the manufacturer’s protocol. Total RNA was extracted from 50–100 mg tissue samples using an RNeasy Lipid Tissue Mini Kit (Qiagen, Germany). The RNA was then treated with DNase I (Qiagen) to eliminate genomic DNA contamination. All RNA isolated was quantified by spectrophotometry using a NanoDrop ND-1000 spectrophotometer (NanoDrop Technologies, USA) and the optical density 260/280 nm ratio was determined; the 260 : 280 ratios were 1.8–2.0. Next, cDNA was prepared from 1 µg of total cellular RNA in a 20 µl reaction volume, using a FirstStrand cDNA synthesis kit and oligo-dT primers (Fermentas, USA). Quantitative assessment of mRNA levels was performed by real-time RT-PCR using an ABI 7500 Fast instrument with Power SYBR Green PCR Master Mix reagent. Real-time conditions were as follows: 95°C (15 s), 40 cycles at 95°C (15 s), and 60°C (1 min). Specificity assessment was done by performing melting curve analysis (60°C to 95°C in temperature ramp melting); only one PCR product was amplified under these conditions. Reaction mixtures contained 10 µl of 2 × SYBR Green supermix, 3 µl of primers (0.4 µmol/l each), 2 µl of cDNA template and 3 µl of water. Each sample was analyzed in two technical replicates, and mean Ct values were used for further analysis. The relative quantity of a target, normalized to the endogenous control Gapdh gene and relative to a calibrator, is expressed as 2–ΔΔCt (-fold difference), where Ct is the threshold cycle, ΔCt = (Ct of target genes) – (Ct of endogenous control gene), and ΔΔCt = (ΔCt of samples for target gene) – (ΔCt of calibrator for the target gene). The following primer pairs were used: for Cat (F: ATG GCT TTT GAC CCA AGC AA; R: CGG CCC TGG AGC ATC TTG T), for Sod1 (F: CAC TCT AAG AAA CAT GGC G; R: CTG AGA GTG AGA TCA CAC G), for Gpx5 (F: CAC CCC TCA GAG ACT GTG GT; R: TTG ACA GTG CTG ACA GGA GC) and for Gsr (F: GGA AGT CAA CGG GAA AAA GTT CAC TG; R: CAA TGTA ACC GGC ACC CAC AAT AAC). The primer sequences used in the study were obtained from the references [37, 46, 47], modified according to the sequence information obtained from the NCBI database, and were synthesized by Oligo.pl (IBB PAN, Poland).
Immunohistochemistry
The dissected epididymides were fixed in Bouin’s fluid (1 h), and then washed with absolute ethanol (3 times over 3 h), absolute ethanol with xylene (1 : 1) (twice over 1 h) and xylene (3 times over 20 min). Then, after 3 h of saturation of the tissues in liquid paraffin, the samples were embedded in paraffin blocks. Using a microtome (Microm HM340E), 3–5 µm serial sections were taken and placed on Polysine microscope slides (Thermo Scientific, UK; cat. no. J2800AMNZ). The sections of the epididymides were deparaffinized in xylene and rehydrated in decreasing concentrations of ethanol, and then used for immunohistochemical staining. In order to expose the epitopes, the sections were boiled twice in 10 nM citrate buffer (pH 6.0) in a microwave oven (700 W for 4 and 3 min). Once cooled and washed with PBS, the endogenous peroxidase was blocked using a 3% solution of perhydrol in methanol, and then the slides were incubated for 60 min at room temperature with primary antibodies against SOD-1 (Santa Cruz Biotechnology, sc-11407), CAT (Santa Cruz Biotechnology, sc-50508), GPX-5 (Santa Cruz Biotechnology, sc-376877) and GR (Santa Cruz Biotechnology, sc-133136). The final dilution of each antibody was 1 : 300. To visualize the antigen-antibody complex, a Dako LSAB+System-HRP was used (Code K0679; DakoCytomation, Glostrup, Denmark), based on the reaction of avidin-biotin-horseradish peroxidase with DAB as a chromogen, according to the included staining procedure instructions. Sections were washed in distilled H2O and counterstained with hematoxylin. For a negative control, specimens were processed in the absence of a primary antibody. Positive staining was determined microscopically (Leica DM5000B, Wetzlar, Germany) by visual identification of brown pigmentation.
Statistical analysis
The results of the qRT-PCR analysis were analyzed using Statistica 6.1 software (StatSoft, Kraków, Poland). The arithmetic means and SDs (x ± SD) were calculated for each parameter. Each variable was tested for normality with the Shapiro-Wilk test. As most of the results deviated from a normal distribution, a nonparametric test was used for further analysis. To assess the differences between the groups, the nonparametric Mann-Whitney U-test was used. A probability p ≤ 0.05 was considered statistically significant. Correlations between the parameters were examined using Spearman’s rank correlation coefficient (rs ).
Results
Epididymis weight increased over time in the offspring in both the F1:Control (rs = +0.86, p = 0.0048) and the F1:Fin (rs = +0.94, p = 0.0048) groups of rats, with significant differences in weight being observed between the groups at PND 14, 28 and 90 (Table I).
Table I
Epididymis weight of rat offspring born from females fertilized by control or finasteride-treated male rats
Age [PND] | Epididymis weight [mg] | % of change vs F1:Control | |
---|---|---|---|
F1:Control | F1:Fin | ||
7 | 12.28 ±3.52 | 12.48 ±1.86 | ↑ 1.6% |
14 | 17.15 ±4.68 | 23.72 ±4.03*** | ↑ 38.3% |
21 | 29.57 ±4.09 | 31.46 ±7.76 | ↑ 6.4% |
28 | 46.87 ±7.69 | 39.83 ±5.96* | ↓ 15.1% |
90 | 492.7 ±31.34 | 577.08 ±42.31*** | ↑ 17.1% |
F1:Control, F1:Fin – rat offspring born from females fertilized by the control or finasteride-treated male rats, respectively. Values express arithmetic means ± SD (n = 5 per age group). Common superscripts within a row denote statistically significant differences between compared groups evaluated by the Mann-Whitney U-test:
Antioxidant enzymes’ mRNA expression in epididymal homogenates
Catalase: A decrease of CAT mRNA expression during aging was observed in both the F1:Control (r s = –0.64, p = 0.000001) and F1:Fin groups (rs = –0.41, p = 0.0036), as shown in Figure 1 A. When comparing the F1:Fin and the F1:Control groups with respect to animal age (Figure 1 B), during the first month of life the transcript level of CAT was statistically significantly lower in the F1:Fin group at PND 28 (more than 31%); in the mature epididymis the level of CAT mRNA was statistically higher in the F1:Fin group (by about 61%).
Figure 1
Representative of catalase mRNA levels (normalized to GAPDH) in homogenates of epididymal tissue of control offspring (F1:Control) and those born from females fertilized by finasteride-treated male rats (F1:Fin). Values are expressed as arithmetic means ± SD; differences were evaluated using the Mann-Whitney U-test (n = 5 per age group). A – Asterisks denote statistically significant differences between successive days of development (PND: postnatal day) in F1:Control and F1:Fin groups: *p < 0.01, **p < 0.001. B – Asterisks denote statistically significant differences between F1:Control and F1:Fin on particular days of development (PND: postnatal day): *p < 0.01. The diagram also shows the percentage of increase/decrease of CAT mRNA in F1:Fin in comparison to F1:Control
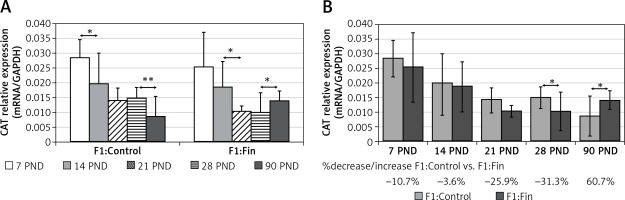
Superoxide dismutase 1: An increase of SOD1 mRNA level with age was observed in both the F1:Control (rs = +0.57, p = 0.00005) and F1:Fin groups (rs = +0.45, p = 0.000005). Figure 2 A shows the comparison of SOD mRNA expression in each age group of the F1:Control and the F1:Fin groups. Comparing the individual days of postnatal development of F1:Fin to the F1:Control group (Figure 2 B), differences in SOD1 mRNA expression were observed at PND 7, 14, 21 and 90 (increase of about 276%, decreases of 37% and 71%, and an increase over 114% for F1:Fin, respectively).
Figure 2
Representative of superoxide dismutase 1 mRNA levels (normalized to GAPDH) in homogenates of epididymal tissue of control offspring (F1:Control) and those born from females fertilized by finasteride-treated male rats (F1:Fin). Values are expressed as arithmetic means ± SD; differences were evaluated using the Mann-Whitney U-test (n = 5 per age group). A – Asterisks denote statistically significant differences between successive days of development (PND: postnatal day) in F1:Control and F1:Fin groups: *p < 0.01, **p < 0.001, ***p < 0.0001. B – Asterisks denote statistically significant differences between F1:Control and F1:Fin on particular days of development (PND: postnatal day): *p < 0.01, **p < 0.001, ***p < 0.0001. The diagram also shows the percentage of increase/decrease of SOD1 mRNA in F1:Fin in comparison to F1:Control
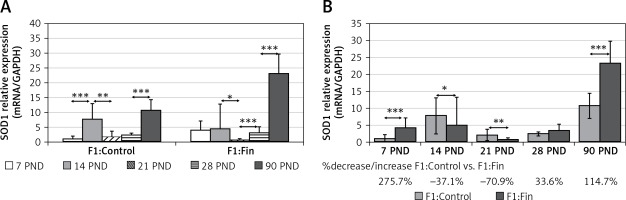
Glutathione peroxidase 5: During postnatal development, a very strong increase in GPX5 mRNA expression was noted in both the F1:Control (rs = +0.80, p = 0.0000001) and F1:Fin (rs = +0.89, p = 0.0000001) groups of rats, as shown in Figure 3 A. Comparing the individual days of postnatal development of the F1:Fin group to the F1:Control group (Figure 3 B), the differences in GPX5 mRNA expressions were statistically significantly higher in the F1:Fin group at PND 14 (2133% increase) and PND 21 (335% increase), with a very strong but not statistically significant decrease at PND 90 (62%).
Figure 3
Representative of glutathione peroxidase 5 mRNA levels (normalized to GAPDH) in homogenates of epididymal tissue of control offspring (F1:Control) and those born from females fertilized by finasteride-treated male rats (F1:Fin). Values are expressed as arithmetic means ± SD; differences were evaluated using the Mann-Whitney U-test (n = 5 per age group). A – Asterisks denote statistically significant differences between successive days of development (PND: postnatal day) in F1:Control and F1:Fin groups: *p < 0.01, **p < 0.001, ***p < 0.0001. B – Asterisks denote statistically significant differences between F1:Control and F1:Fin on a particular day of development (PND: postnatal day): **p < 0.001, ***p < 0.0001. The diagram also shows the percentage of increase/decrease of GPX5 mRNA in F1:Fin in comparison to F1:Control
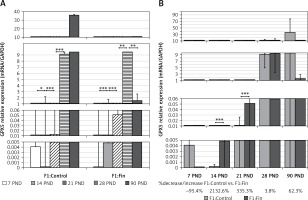
Glutathione reductase: There was no visible decreasing/increasing trend for GR mRNA with age (F1:Control: rs = –0.058, p = 0.67; F1:Fin: rs = –0.027, p = 0.85). However, there were statistically significant differences in transcript levels at different age group (Figure 4 A). Comparing the individual days of postnatal development of the F1:Fin group to the F1:Control group (Figure 4 B), a statistically significant decrease in GR mRNA expression was observed at both PND 21 and 28 (74% and 10%, respectively).
Figure 4
Representative of glutathione reductase mRNA levels (normalized to GAPDH) in homogenates of epididymal tissue of control offspring (F1:Control) and those born from females fertilized by finasteride-treated male rats (F1:Fin). Values are expressed as arithmetic means ± SD; differences were evaluated using the Mann-Whitney U-test (n = 5 per age group). A – Asterisks denote statistically significant differences between successive days of development (PND: postnatal day) in F1:Control and F1:Fin groups: *p < 0.01, **p < 0.001. B – Asterisks denote statistically significant differences between F1:Control and F1:Fin on a particular day of development (PND: postnatal day): ***p < 0.0001. The diagram also shows the percentage of increase/decrease of GR mRNA in F1:Fin in comparison to F1:Control
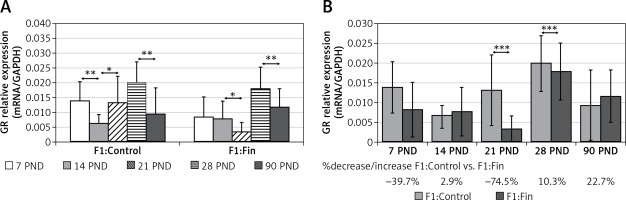
Immunohistochemical expression and localization of antioxidant enzymes in the epididymis
The gradient of staining intensity is described according to the adopted scale in Table II.
Table II
Antioxidant immunoreactivity based on visual examination of intensity
Age [PND] | CAT | SOD 1 | GPX 5 | GR | ||||
---|---|---|---|---|---|---|---|---|
F1:Control | F1:Fin | F1:Control | F1:Fin | F1:Control | F1:Fin | F1:Control | F1:Fin | |
7 | +++ | ++ | ++ | ++ | +/– | +/– | ++ | + |
14 | ++ | ++ | ++ | ++ | +/– | +/– | ++ | ++ |
21 | + | + | + | +/– | + | + | + | +/– |
28 | + | + | ++ | ++ | ++ | ++ | ++ | + |
90 | +/– | ++ | +++ | +++ | +++ | ++ | +/– | + |
Catalase: The immunoexpression of CAT in the control rats during the first month of postnatal life (Figures 5 A‒D) gradually decreased and had a more apical localization (red arrows) or granular across the cytoplasm, in contrast to the mature epididymis (Figure 5 E), which exhibited an almost negative but basal (black arrows) or diffuse pattern across the whole cytoplasm (white asterisk), with many epithelial cells immunonegative (blue arrow).
Figure 5
Immunoexpression of catalase within the epididymis of rat offspring born from females fertilized by control (A–E) and finasteride-administered (F–J) male rats. Well-visible immunoexpression of CAT in epididymal epithelial cells: during the first month of development (A–D, F–I) mainly in the supranuclear, apical region of cells; after reaching maturity (E, J), mainly basal localization. Objective magnification: A‒J ×100, scale bar: 20 μm. In the upper right corner of the J photograph the negative control of IHC reaction. F1:Control, F1:Fin – rat offspring born from females fertilized by the control or finasteride-treated male rats, respectively (n = 5 per age group); PND – postnatal day; red arrows – apical immunoexpression of enzyme; black arrows – basal/perinuclear immunoexpression of enzyme; blue arrow – immunonegative cell; white asterisk – diffuse/cytoplasmic immunoexpression
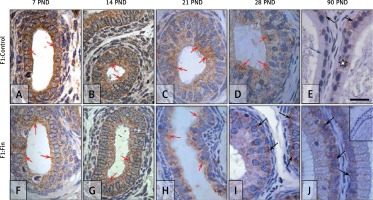
In the F1:Fin group of rats an apical localization (red arrows) of catalase was visible during the first 3 weeks of development (Figures 5 F‒H), taking place more basally (black arrows) in epididymal epithelial cells at PND 28 (Figure 5 I) and PND 90 (Figures 5 J). In immature epididymis of F1:Fin rats (Figures 5 F‒I), CAT expression was lower than in the control (Figures 5 A‒D), in contrast to the mature tissues, where it was higher (Figure 5 J).
Superoxide dismutase 1: In the F1:Control group the SOD1 staining intensity had a fluctuating pattern ‒ moderate during the first, second and fourth week of life (Figures 6 A, B, D), weak at PND 21 (Figure 6 C), and highest in the mature epididymis (Figure 6 E). In immature epididymis (Figures 6 A‒D) SOD1 was mainly localized in apical regions of epithelial cells (red arrows); at PND 90 (Figure 6 E) most cells had the whole cytoplasm immunopositive (white asterisk), while some were immunonegative (blue arrow).
Figure 6
Immunoexpression of superoxide dismutase 1 within the epididymis of rat offspring born from females fertilized by control (A–E) and finasteride-administered (F–J) male rats. Strong, homogeneous immunolocation of SOD1 in epididymal epithelial cells during first 2 weeks of life (A–B, F–G); quenched but not different in respect of location and type of cells immunoexpression on 21st and 28th day of life (C–D, H–I); diffuse cytoplasmic or perinuclear expression during maturity (E, J). Objective magnification: A‒J 100×, scale bar: 20 μm. In the upper right corner of the J photograph the negative control of IHC reaction. F1:Control, F1:Fin – rat offspring born from females fertilized by the control or finasteride-treated male rats, respectively (n = 5 per age group); PND – postnatal day; red arrows – apical immunoexpression of enzyme; black arrows – basal/perinuclear immunoexpression of enzyme; blue arrow – immunonegative cell; white asterisk – diffuse/cytoplasmic immunoexpression
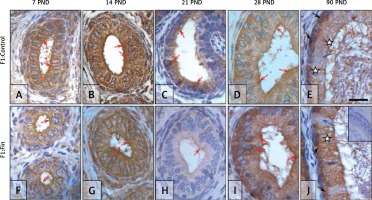
In the F1:Fin groups, the intensity and localization of SOD1 also depended on age. Intensity had the lowest level at PND 21 (Figure 6 H); apical localization (red arrows) was observed during the pre-pubertal period (Figures 6 F‒H); and in the mature epididymis (Figure 6 J) the majority of epithelial cells had a moderate, diffused (white asterisk) or strong perinuclear (black arrows) SOD1 reaction.
Glutathione peroxidase 5: Immunoexpression of GPX5 during the first 3 weeks of postnatal life was very weak in both the F1:Control (Figures 7 A‒C) and F1:Fin (Figures 7 F‒H) groups of rats. At PND 28 in the F1:Control rats (Figure 7 D), some epithelial cells expressed GPX5 across the cytoplasm (white asterisks) while others had an immunopositive apex (red arrows). GPX5 immunoexpression in the epididymis of adult animals (Figure 7 E) became very strong and was located within some cell cytoplasm (white asterisk), apical areas of cells (red arrow) or in stereocilia (pink arrows); spermatozoa (yellow asterisk) located within the lumen of ductus epididymis were also GPX5-reactive.
Figure 7
Immunoexpression of glutathione peroxidase 5 within the epididymis of rat offspring born from females fertilized by control (A–E) and finasteride-administered (F–J) male rats. Almost invisible, very weak GPX5 expression in epididymal epithelial cells during first three weeks of life (A–C, F–H); the severity of the reaction in the individual cells on 28th day of life (D, I); and during maturity GPX5 become stronger mainly in F1:Control rats (E). Objective magnification: A‒J 100×, scale bar: 20 μm. In the upper right corner of the J photograph the negative control of IHC reaction. F1:Control, F1:Fin – rat offspring born from females fertilized by the control or finasteride-treated male rats, respectively (n = 5 per age group); PND – postnatal day; red arrows – apical immunoexpression of enzyme; pink arrows – immunopositive stereocilia; white asterisk – diffuse/cytoplasmic immunoexpression; yellow asterisk – immunopositive spermatozoa
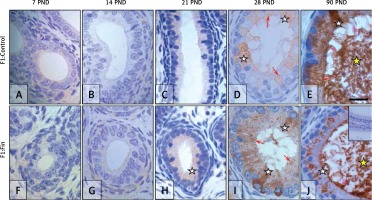
The same mode of immunoexpression and immunolocalization was observed in F1:Fin rats (Figures 7 F–J), but signaling in mature F1:Fin rats (Figure 7 J) was weaker than in F1:Control (Figure 7 E) rats.
Glutathione reductase: The expression of GR in epididymal epithelial cells during the first 3 weeks in the F1:Control group (Figures 8 A–C) displayed a cytoplasmic diffuse pattern (white asterisks) with a stronger indication in the apical part of the cells (red arrows). At PND 28 (Figure 8 D), GR exhibited a strong diffuse expression (white asterisk) with tight indication around the nucleus (black arrows). Within the mature epididymis (Figure 8 E) GR expression was much lower than in the immature cells (Figures 8 A–D) and mainly located basally, around the cell nucleus (black arrows), but some cells were immunonegative (blue arrows).
Figure 8
Immunoexpression of glutathione reductase within the epididymis of rat offspring born from females fertilized by control (A–E) and finasteride-administered (F–J) male rats. During the first 2 weeks of development the localization and intensity of GR expression is comparable in F1:Control and F1:Fin (A–B, F–G); much lower relative to control (C), almost invisible immunoreaction in PND 28 F1:Fin (H); strong, diffuse pattern of IHC in PND 28 F1:Control (D) and lower in PND 28 F1:Fin (I); during puberty (E, J) the expression became much lower than in the prepubertal period. Objective magnification: A‒J 100×, scale bar: 20 μm. In the upper right corner of the J photograph the negative control of IHC reaction. F1:Control, F1:Fin – rat offspring born from females fertilized by the control or finasteride-treated male rats, respectively (n = 5 per age group); PND – postnatal day; red arrows – apical immunoexpression of enzyme; black arrows – basal/perinuclear immunoexpression of enzyme; blue arrows – immunonegative cells; white asterisk – diffuse/cytoplasmic immunoexpression
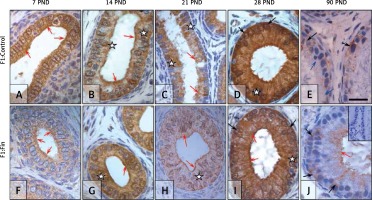
In the F1:Fin group, the intensity and localization of GR depended on age. During the first 2 weeks of life (Figures 8 F, G) GR was expressed across the cell cytoplasm (white asterisk), in particular at the cell apex (red arrows). At PND 21 immunostaining in the F1:Fin group became low (Figure 8 H); 1 week later (Figure 8 I) it became moderate and diffuse (white asterisk) and some cells had apical (red arrows) or perinuclear (black arrows) localization. At PND 90 (Figure 8 J), some epithelial cells had a moderate GR-positive reaction in the nuclear (black arrows) or apical (red arrow) region of the cell. Staining intensity in immature epididymis in the F1:Fin group (Figures 8 F‒I) was lower than in the F1:Control rats (Figures 8 A‒D), but in the mature rats (Figure 8 J) GR-immunoexpression in some cells was higher than in the control (Figure 8 E).
Correlation of mRNAs of antioxidant enzymes with serum T and DHT
Table III shows the blood serum level of androgens presented in our previous study [38], which was used to make correlations with the levels of antioxidant enzyme mRNA.
Table III
Testosterone and dihydrotestosterone concentrations in blood serum of rat offspring born from females fertilized by control (F1:Control) or finasteride-treated (F1:Fin) male rats (published in [38])
Age [PND] | T [ng/mg] | DHT [pg/mg] | ||
---|---|---|---|---|
F1:Control | F1:Fin | F1:Control | F1:Fin | |
14 | 3.91 ±0.16 | 1.68 ±1.03** | 1608.65 ±75.24 | 774.81 ±46.07** |
21 | 2.62 ±0.29 | 2.67 ±0.21 | 1096.51 ±137.78 | 1053.89 ±71.04 |
22 | 2.90 ±0.51 | 3.46 ±0.59 | 1189.19 ±261.44 | 1577.09 ±189.19** |
28 | 2.86 ±0.15 | 3.52 ±0.52** | 1322.47 ±41.01 | 1482.14 ±26.40 |
90 | 0.46 ±0.11 | 0.92 ±0.61*** | 443.64 ±180.51 | 280.60 ±25.83** |
There were statistically significant correlations between the concentrations of androgens and mRNA expression for CAT and GPX5 only (Table IV).
Table IV
Correlation between serum androgens (T, DHT) and mRNAs for catalase, superoxide dismutase 1, glutathione peroxidase 5 and glutathione reductase in epididymal homogenates of male control rats offspring (F1:Control) and finasteride-treated male rat offspring (F1:Fin)
With respect to age in the F1:Control group a moderate positive correlation between DHT and CAT mRNA was observed. In contrast, in the F1:Fin group a moderate negative correlation was observed for both androgens (T and DHT) and CAT mRNA. Age-dependent strong negative correlations between GPX5 mRNA and both androgens (T, DHT) were observed just in the F1:Control group.
Discussion
The functions of the epididymis and thus post-testicular spermatozoa maturation are androgen-dependent [48–50]. To acquire a proper physiology the male gametes require interaction with the epididymal luminal fluid [51], rich in antioxidant enzymes [16]. Nevertheless, a physiological level of ROS is necessary for suitable DNA condensation, sperm hyperactivation, forward motility, capacitation and acrosome reaction [11–13]. Consequently, spermatozoa have to face the so-called ‘oxygen paradox’ [11, 52, 53] and any agents that disturb the epididymal functions may also affect the correct and required levels of antioxidant enzymes [13].
Weight of epididymides
In this study we observed differences in the weight of the epididymides of rats born from females fertilized by finasteride-treated male rats in comparison to the F1:Control animals. Due to the fact that finasteride, as an inhibitor of 5α-reductase, altered androgen homeostasis, as shown in a previous work [38], it could be considered an endocrine disruptor (ED). A reduction in the size of the epididymides in male offspring was induced by the treatment of mice during pregnancy with bisphenol A (BSA; ED with estrogenic activity) [54]. The organ’s mass has also been reduced by the postnatal influence of dioxin [55], nonylphenol [56], BSA [57], lindane (an insecticide with estrogenic and antiestrogenic properties) [58], methoxychlor (a pesticide with estrogenic/antiandrogenic activity) [59] and atrazine (a herbicide that has endocrine-disrupting effects on the reproductive system) [60]. In this study, the weights of the epididymides from the F1:Fin rats were higher than the control animals of the same age (apart from PND 28 – similar testis weight [38]), probably because of a correlation with total body weight or the same weight fluctuation differences in the testes [38]. These observations may be associated with the modulation of adipose tissue metabolism by androgens [61], and therefore could be affected by changes in the T/DHT ratio observed in our experiment.
Age-related changes in expression of antioxidant enzymes in the epididymis and consequences
The results of this study confirm the changes in antioxidant enzyme expression of mRNA and proteins during postnatal maturation, being different in immature rats (PND 7, 14, 21, 28) than in mature animals (PND 90), in agreement with other reports [62] that demonstrated changes in antioxidant enzymes’ (GPX, GR, CAT, SOD) activity in male reproductive tissues (such as the cauda epididymidis) in young (4-month-old) rats when compared to older (21-month-old) rats. Some authors [16] consider CAT one of the most controversial enzymes within the epididymis, such as when CAT mRNA expression is extremely low, undermining its potential role as a major antioxidant in the tissue. It has been reported that the role of CAT only appears important in concentrations of H2O2 higher than 10–6 M [63], yet these concentrations in normal physiological conditions are rarely achieved besides an oxidative burst or over-expression of SOD [64]. According to Vernet et al. [16], CAT does not appear to be a major participant in the control of oxidative stress within the epididymis, yet other researchers [65, 66] have demonstrated that catalase activity was associated with low sperm quality; in the light of these reports, the higher level of CAT in adult F1:Fin rats in the present study may have a negative impact on the fertility of offspring of finasteride-treated male rats.
The copper/zinc form of SOD (SOD1) catalyzes the dismutation of O2 to generate H2O2 that could potentially damage the epididymis and spermatozoa [16]. Seminal SOD activity is positively associated with sperm concentration and their overall motility, whereas it is inversely associated with sperm DNA fragmentation [19]. The very high expression of SOD1 mRNA in the adult F1:Fin rats in the present study could affect epididymal sperm maturation. In light of this danger caused by SODs, the presence of the epididymal secretory form of glutathione peroxidase (GPX5) which covers the spermatozoa head is noticeable [16, 28]. It has been demonstrated that GPX5 protects cells from oxidative stress induced lipid peroxidation and DNA mutation [67], maintains sperm DNA integrity [68] and regulates the synthesis of prostaglandin, key signaling molecules needed by spermatozoa [69]. Therefore, the decrease of GPX5 in the adult F1:Fin rats observed in our study could result in inadequate spermatozoa protection and subsequent dysfunction. In the reproductive system there is also rich expression of glutathione reductase, which supports spermatogenesis and protects the sulfhydryl groups and unsaturated fatty acids of spermatozoa plasmalemma against peroxidation during epididymal maturation and storage [70]. In our study we observed a reduction in GR transcript at the end of the first month in the F1:Fin group and a level not statistically higher in mature rats than controls. Consequently, it may lead to a lesion of the plasma membrane of spermatozoa and its insufficient fluidity.
The different levels of antioxidant enzymes in the successive days of development could be correlated with postnatal formation of the blood-epididymis barrier. An electron microscopic study by Agrawal and Hoffer [71] showed gradual postnatal development of the blood-epididymis barrier with formation complete by day 21. The various levels of antioxidant enzymes in the epididymis could be connected with the ongoing processes in the testes, such as intense cell proliferation/apoptosis to provide the proper ratio between the number of Sertoli and spermatogonial stem cells, blood-testis-barrier formation, and the secretory function undertake by Sertoli cells during the first wave of spermatogenesis [72–78]. Or there may be just a correlation with the changes in antioxidant levels in the testes during life, as was documented by Samanta et al. [79].
Androgens as regulators of antioxidant enzymes in epididymides
The different levels of antioxidant enzymes in the successive days of development could be correlated with the hormonal secretion of testicular Leydig cells, which change over the animal’s life (plasma testosterone concentration in pubertal rats is higher than in adults) [80–82], which is in conformity with our observation. Moreover, the specific regions of the epididymis (caput, corpus, cauda) possess distinct spatially restricted gene expression profiles, ensuring suitable epididymal functions essential to the different steps of sperm maturation, where factors such as steroidal hormones can affect the pattern of gene expression [83]. It has been confirmed that some genes encoding antioxidant enzymes are androgen-dependent [24, 30–33, 35]. Our study revealed an age-dependent correlation between serum androgen concentration and various antioxidant enzyme transcription levels. These correlations when comparing F1:Control to F1:Fin rats were different. Catalase mRNA positively and moderately correlated with DHT in control rat offspring, but in finasteride-treated male rat offspring the correlation was moderate and negative for both androgens (T, DHT). Only in the F1:Control group were there strong negative correlations between GPX5 mRNA and the androgens (both T and DHT). Other enzymes’ transcripts (SOD1, GR) were not linked to serum androgens concentration. Consequently, the transgenerational effect of finasteride could alter the antioxidant defense status of the epididymis.
Endocrine disruptors and changes in epididymal antioxidant enzymes
There are many reports indicating the adverse influence of endocrine disruptors [55, 56] such as dioxin [55], pesticides [59] or insecticides [58] with hormonal properties, on a decrease of SOD, CAT, GR and GPX activity in sperm collected from the epididymis. Significantly decreased activities of these enzymes have been documented in epididymis homogenates from bisphenol A-treated rats [84] and lindane-treated rats, which had more significant changes in the cauda than the caput or corpus regions of the organ [58]. Methoxychlor similarly decreased the activity of antioxidant enzymes (SOD, CAT, GR, GPX) in the epididymis with respect to the regions of the organ [59]. The study by Kolasa et al. [37] demonstrated that finasteride treatment of adult rats changed the epididymal region-specific immunoexpression of antioxidant enzymes (GPX5: increase in the caput of treated rats compared to control rats; E-SOD: increase in the caput and decrease in the cauda of treated rats). Also, treatment of Wistar male rats with ethanol and atrazine showed diverse results: CAT activity in epididymal homogenates decreased, SOD and GPX activity increased [60]. The action of finasteride could be compared to that mentioned above of EDs with estrogenic/antiandrogenic activity, as according to the official brochure from the manufacturer of Propecia (Merck Sharp & Dohme Corporation, Kenilworth, USA) [85], “mean circulating levels of testosterone and estradiol increased by approximately 15%”. Additionally, the study by Vaughan et al. [86] established that finasteride treatment (1 mg/day) increased the concentration of testosterone and led to its conversion to estradiol, and finally resulted in increased levels of estrogen.
The different levels of plasma and intratesticular levels of T and DHT in control rats (F1:Control) when compared to F1:Fin animals [38] may reflect the fluctuation/oscillation of antioxidant expression in the successive days of the experiment. In general, other male reproductive toxins such as chlorpyrifos (insecticide) [87], atrazine (pesticide) [60], and nonylphenol (ED) [56], that postnatally influence an organism also caused a reduction in plasma testosterone concentration. Similarly, the inhibition of 5α-reductase 2 activity by finasteride changed plasma androgen concentrations in finasteride-treated adult male rats [37] and in male offspring of these rats [38]. The differences in androgen levels between the F1:Fin and F1:Control groups of rats exhibit some resemblance to the results of studies which explored prenatal exposure to anti-androgenic endocrine disruptors such as flutamide [88] or vinclozolin [89] that reduced serum testosterone concentrations in adult animals. But in our data, the adult (PND 90) offspring of finasteride-treated male rats had an increased T serum level and decreased DHT serum level. Anyway, there is a lot of evidence for considering the role of DHT-dependent mechanisms in controlling epididymal functions [90].
In conclusion, finasteride treatment of parental rats resulted in changes in their offspring, namely: (i) antioxidant enzyme (CAT, SOD1, GR, GPX5) expression in the epididymis at mRNA and protein levels, (ii) immunolocalization of the enzymes in epididymal epithelial cells, (iii) correlations (positive/negative/lack) between serum androgens and transcripts of antioxidant enzymes (CAT, GPX5) in finasteride-treated male offspring in comparison to controls.
These mentioned changes could disturb the epididymal microenvironment, such as the antioxidant defense system and concentration of ROS, which are needed for correct post-testicular spermatozoa maturation.